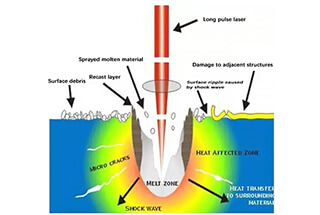
Ever wondered how a tiny semiconductor can produce powerful laser light? Semiconductor lasers, pivotal in technologies from fiber optics to medical devices, have evolved dramatically since their inception in 1962. This article explores their working principles, historical milestones, and diverse applications. Discover how semiconductor lasers work, the materials involved, and their groundbreaking impact on modern technology. Dive in to understand their journey from basic science to being an essential part of everyday tech!
Since the invention of the world’s first semiconductor laser in 1962, semiconductor lasers have undergone tremendous changes, greatly promoting the development of other sciences and technologies, and are considered one of the greatest human inventions of the twentieth century.
In recent decades, the development of semiconductor lasers has been even more rapid, making it one of the fastest-growing laser technologies in the world.
The application of semiconductor lasers covers the entire field of optoelectronics and has become the core technology of optoelectronics science today.
Due to the advantages of small size, simple structure, low input energy, long life, easy modulation, and low price of semiconductor lasers, they are now widely used in the field of optoelectronics and are highly valued by countries around the world.
The semiconductor laser is a miniaturized laser with Pn junction or Pin junction composed of direct band gap semiconductor material as the working material.
There are dozens of semiconductor laser working substances, and the semiconductor materials that have been made into lasers include gallium arsenide, indium arsenide, indium antimonide, cadmium sulfide, cadmium telluride, lead selenide, lead telluride, aluminum gallium arsenic, indium phosphorus arsenic, etc.
There are three main excitation methods for semiconductor lasers, ie:
Most semiconductor lasers are excited by electrical injection, meaning that a forward voltage is applied to the Pn junction to produce excited emission in the junction plane region, which is a forward-biased diode.
Therefore, the semiconductor laser is also called a semiconductor laser diode.
For semiconductors, since the electrons jump between energy bands rather than between discrete energy levels, the jump energy is not a definite value, which causes the output wavelength of semiconductor lasers to spread over a wide range.
They emit wavelengths in the range of 0.3 to 34 μm.
The wavelength range is determined by the energy band gap of the material used, and the most common is the AlGaAs double heterojunction laser with an output wavelength of 750 to 890 nm.
Schematic diagram of the laser structure
The manufacturing technology of semiconductor lasers has gone through various processes from diffusion to liquid phase epitaxy (LPE), vapor phase epitaxy (VPE), molecular beam epitaxy (MBE), MOCVD method (metal organic compound vapor deposition), chemical beam epitaxy (CBE) and various combinations of them.
The biggest drawback of semiconductor lasers is that the laser performance is greatly affected by temperature, and the divergence angle of the beam is large (generally between a few degrees and 20 degrees), which results in poor directionality, monochromaticity and coherence.
However, with the rapid development of science and technology, research on semiconductor lasers is advancing in the direction of depth, and the performance of semiconductor lasers is constantly improving.
Semiconductor lasers, as the core of semiconductor optoelectronics technology in the 21st century information society, will make greater progress and play a greater role.
The semiconductor laser is a coherent radiation source, to enable it to produce laser light, there must be three basic conditions :
1. Gain condition
To establish the inversion distribution of carriers in the excitation medium (active region), the electron energy in a semiconductor is represented by a series of energy bands consisting of a series of nearly continuous energy levels.
Therefore, to achieve particle number inversion in semiconductors, it is necessary to be in between two energy band regions.
The number of electrons at the bottom of the conduction band in the higher energy state is much larger than the number of holes at the top of the valence band in the lower energy state. This is achieved by adding forward bias to the homojunction or heterojunction and injecting the necessary carriers into the active layer to excite the electrons from the lower energy valence band to the higher energy conduction band.
Excited emission occurs when a large number of electrons in the particle number reversal state combine with holes.
2. To actually obtain the relevant stimulated radiation
To achieve multiple feedback and the formation of laser oscillation, excited radiation must be made in the optical resonant cavity.
The resonant cavity of a laser is formed by using the natural surface solution of a semiconductor crystal as a reflector, typically with a highly reflective multilayer dielectric film on the non-emitting end and a partially reflective film on the emitting side.
In the case of F-p cavity (Fabry-Perot cavity) semiconductor lasers, the F-p cavity can be easily formed by using the natural solution plane of the crystal perpendicular to the p-n junction plane.
3. In order to form stable oscillations, the laser medium must be able to provide a large enough gain
To compensate for the optical loss caused by the resonant cavity and the loss caused by the laser output from the cavity surface, it is necessary to constantly increase the optical field in the cavity.
This requires a strong enough current injection, that is, sufficient particle number reversal. The higher the degree of particle number reversal, the greater the gain obtained, so it is necessary to meet a certain current threshold condition.
When the laser reaches the threshold value, light with a specific wavelength can resonate in the cavity and be amplified, eventually forming a laser and continuously outputting.
It can be seen that in semiconductor lasers, the dipole leap of electrons and holes is the basic light emission and light amplification process.
For new semiconductor lasers, it is now recognized that quantum wells are the fundamental driving force for the development of semiconductor lasers.
The topic of whether quantum wires and quantum dots can take full advantage of quantum effects has been extended into this century, and scientists have tried to make quantum dots in various materials with self-organized structures, while GaInN quantum dots have been used in semiconductor lasers.
Semiconductor lasers were first developed in the early 1960s as homogeneous junction lasers, which were pn junction diodes made on a single material. When subjected to a high forward current injection, electrons were continuously injected into the p region, and holes were continuously injected into the n region, resulting in a reversal of the carrier distribution in the original pn junction depletion zone. Since the electron migration rate is faster than the hole migration rate, the emission of radiation and compound particles occurs in the active zone, emitting fluorescence, and under certain conditions, a pulse-form semiconductor laser occurs.
The second stage of semiconductor laser development is the heterostructure semiconductor laser, which consists of two different bandgap semiconductor material thin layers, such as GaAs and GaAlAs. The first of these was a single heterostructure laser (1969). Single heterojunction injection lasers (SHLD) within the p-zone of GaAsP-N junction to reduce the threshold current density, the value of which is an order of magnitude lower than that of homojunction lasers, but single heterojunction lasers still cannot operate continuously at room temperature.
Since the late 1970s, semiconductor lasers have clearly developed in two directions. One is the development of information-based lasers for the purpose of transmitting information, and the other is the development of power-based lasers for the purpose of increasing optical power. This was driven by applications such as pumped solid-state lasers, and high-power semiconductor lasers (continuous output power of 100mw or more, pulsed output power of 5W or more) are now considered high-power semiconductor lasers.
In the 1990s, there was a breakthrough in semiconductor laser technology marked by a significant increase in the output power of semiconductor lasers. Kilowatt-class high-power semiconductor lasers were commercialized, and the output of domestic sample devices reached 600W. Laser wavelengths also expanded from infrared semiconductor lasers to 670nm red semiconductor lasers, followed by the introduction of wavelengths of 650nm, 635nm, blue-green, and blue semiconductor lasers. 10mW-scale violet and even ultraviolet semiconductor lasers were also successfully developed.
In the late 1990s, the development of surface-emitting lasers and vertical-cavity surface-emitting lasers has been considered for a variety of applications in ultra-parallel optoelectronics. Devices at 980 nm, 850 nm, and 780 nm have been made practical in optical systems. Currently, vertical cavity surface emitting lasers are used in high-speed networks for gigabit Ethernet.
Semiconductor lasers are a class of lasers that matured earlier and progressed faster due to their wide wavelength range, simple production, low cost, easy mass production, small size, light weight, and long life. Therefore, their development has been rapid, and the range of applications has now exceeded 300 kinds.
(1) Fiber optic communication:
Semiconductor lasers are the only practical light source for fiber optic communication systems, and fiber optic communication has become the mainstream of contemporary communication technology.
(2) Optical disc access:
Semiconductor lasers have been used for optical disc memory, and their biggest advantage is the large amount of stored sound, text, and graphic information. The use of blue and green lasers can greatly improve the storage density of optical discs.
(3) Spectral analysis:
Far-infrared tunable semiconductor lasers have been used for environmental gas analysis, monitoring atmospheric pollution, automobile exhaust, etc. In the industry, it can be used to monitor the process of vapor-phase precipitation.
(4) Optical information processing:
Semiconductor lasers have been used in optical information management systems. Surface emitting semiconductor laser 2D arrays are ideal light sources for optical parallel processing systems and will be used in computers and optical neural networks.
(5) Laser microfabrication:
Q-switched semiconductor lasers produce high-energy ultra-short light strokes for cutting and punching of integrated circuits.
(6) Laser alarm:
Semiconductor laser alarms are used for a wide range of applications, including burglar alarms, water level alarms, car distance alarms, etc.
(7) Laser printers:
High-power semiconductor lasers have been used in laser printers. The use of blue and green lasers can greatly improve the printing speed and resolution.
(8) Laser barcode scanner:
Semiconductor laser barcode scanners have been widely used for merchandising, as well as for book and file management.
(9) Pumped solid-state lasers:
This is an important application of high-power semiconductor lasers, using them to replace the original atmosphere lamp, can constitute an all-solid-state laser system.
(10) High definition laser TV:
In the near future, semiconductor laser TVs without cathode ray tubes can be put on the market, which utilize red, blue, and green lasers and are estimated to consume 20% less power than existing TV sets.
(1) Laser surgery treatment
Semiconductor lasers have been used for soft tissue excision, tissue joining, coagulation, and vaporization. They have been widely used in general surgery, plastic surgery, dermatology, urology, obstetrics, and gynecology.
(2) Laser kinetic treatment
Photosensitive substances with an affinity for tumors are selectively gathered in cancerous tissues and irradiated by a semiconductor laser to produce reactive oxygen species in cancerous tissues, aiming at necrosis without any damage to healthy tissues.
(3) Life science research
The use of semiconductor laser “optical tweezers,” which can capture live cells or chromosomes and move them to any location, has been used to promote cell synthesis, cell interaction, and other research, as well as a diagnostic technique for forensic science.