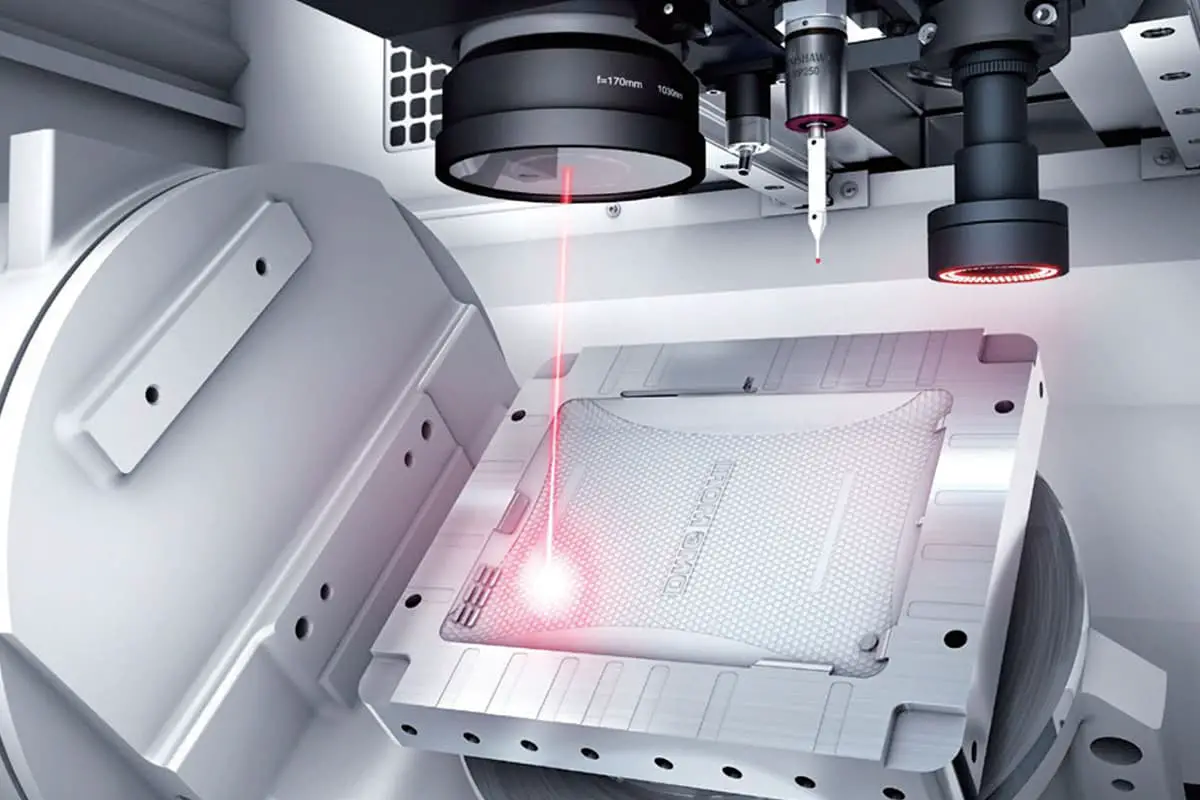
Have you ever wondered how lasers revolutionized everything from surgery to cutting metal? This article dives into the fascinating world of CO2 lasers, explaining their principles, structure, and diverse applications. By reading, you’ll uncover how these powerful tools work and their impact on various industries. Get ready to explore the marvels of CO2 laser technology!
In 1964, Patel obtained continuous laser output at wavelengths near 10.4 microns and 9.4 microns in CO2 gas discharge, giving birth to the world’s first CO2 molecular laser.
It has a significant power and high energy conversion efficiency.
It utilizes the transition between the vibrational-rotational energy levels of CO2 molecules, which results in a rich spectrum. There are dozens of spectral lines for laser output near 10 microns. Its extensive application in industry, military, medical, and scientific research has brought many conveniences to our lives.
In 1966, the aerodynamic CO2 laser was born, drawing great attention to the CO2 laser technology. The introduction of aerodynamics in laser technology has opened up broad prospects for the use of CO2 lasers.
With the advancement of science and technology, laser technology around the world has also developed accordingly. The carbon dioxide laser is currently one of the lasers with high continuous output power. Its early development and mature commercial products have been widely used in fields such as material processing, medical use, military weapons, and environmental measurement.
In the development and application of lasers, the creation and application of CO2 lasers came earlier and more frequently. As early as the late 1970s, CO2 lasers were directly imported from abroad for industrial processing and medical applications.
Since the late 1980s, CO2 lasers have been widely introduced and applied in the field of material processing.
This article mainly introduces the basic principles and structure of the CO2 laser, and focuses on the application of the CO2 laser from three aspects. Finally, it introduces the current research status and future prospects of the CO2 laser.
The production of lasers requires three conditions:
(1) A gain medium that provides amplification as laser working material, and its activated particles (atoms, molecules, or ions) have an energy level structure suitable for stimulated emission;
(2) An external excitation source that pumps particles from lower energy levels to higher ones, causing a particle number inversion between the laser’s upper and lower energy levels;
(3) An optical resonator that extends the working length of the activated medium, controls the direction of the light beam, and selects the frequency of the stimulated emission light to improve monochromaticity.
Compared to ordinary light sources, lasers have four main features: excellent directionality, extremely high brightness, good monochromaticity, and high coherence.
A laser device is a mechanism capable of emitting lasers. The first microwave quantum amplifier was made in 1954, producing a highly coherent microwave beam.
In 1958, A.L. Schawlow and C.H. Townes extended the principles of microwave quantum amplifiers to the optical frequency range and outlined the methods for generating lasers.
In 1960, T.H. Maiman and his team constructed the first ruby laser. In 1961, a helium-neon laser was produced by A. Javan and others, and in 1962, R.N. Hall and his team created a gallium arsenide semiconductor laser. Since then, the types of lasers have been continually expanding.
Apart from free-electron lasers, the fundamental working principles of various lasers are identical.
The essential conditions for producing a laser are particle number inversion and the gain exceeding the loss, so the indispensable components of a system include an excitation (or pump) source and a working medium with metastable energy levels.
Excitation is the excitation state after the working medium absorbs external energy, creating conditions for achieving and maintaining particle number inversion. Excitation methods include optical excitation, electric excitation, chemical excitation, and nuclear energy excitation.
The working medium having a metastable energy level allows stimulated radiation to dominate, thereby achieving light amplification. Common components in a laser device also include a resonant cavity.
However, the resonant cavity (see optical resonant cavity) is not an essential component. The resonant cavity can align the frequency, phase, and direction of the photons within the cavity, thus providing the laser with excellent directionality and coherence.
Moreover, it can effectively shorten the length of the working material and adjust the mode of the laser produced by changing the length of the resonant cavity. Therefore, most laser devices have a resonant cavity.
There are many types of lasers. Below, we will categorize and introduce them based on the laser working material, excitation method, and operation mode.
(1) By working material
Lasers can be grouped into several categories based on the state of the working material:
① Solid (crystal and glass) lasers;
② Gas lasers, further divided into atomic gas lasers, ion gas lasers, molecular gas lasers, and quasi-molecular gas lasers;
③ Liquid lasers, the working materials of which primarily include two types: organic fluorescent dye solutions and inorganic compound solutions containing rare earth metal ions;
④ Semiconductor lasers;
⑤ Free-electron lasers.
(2) By excitation method
① Optically pumped lasers;
② Electrically excited lasers;
③ Chemical lasers;
④ Nuclear pumped lasers.
(3) By operation mode
Due to the different working materials, excitation methods, and application purposes of lasers, their operation modes and working states also vary. They can be divided into several main types:
① Continuous lasers;
② Single-pulse lasers;
③ Repetitive pulse lasers;
④ Modulated lasers;
⑤ Mode-locked lasers;
⑥ Single-mode and frequency-stable lasers;
⑦ Tunable lasers.
As shown in Figure 1, a typical CO2 laser structure is represented. The two mirrors that form the resonant cavity of the CO2 laser are placed on an adjustable cavity rack. The simplest method is to directly affix the mirrors to both ends of the discharge tube.
Basic Structure:
① Laser Tube
This is the most critical part of the laser. It is usually made up of three parts (as shown in Figure 1): the discharge space (discharge tube), the water cooling jacket (tube), and the gas reservoir.
The discharge tube is typically made from hard glass and often employs a cascading cylinder structure. It affects the output of the laser and the power of the laser output. The length of the discharge tube is proportional to the output power.
Within a certain length range, the power output per meter of discharge tube increases with the total length.
Generally speaking, the thickness of the discharge tube has no effect on the output power. The water-cooling jacket tube, like the discharge tube, is made from hard glass.
Its function is to cool the working gas, stabilizing the output power. The gas storage tube is connected to both ends of the discharge tube, meaning one end of the gas storage tube has a small hole connected to the discharge tube, and the other end is connected to the discharge tube through a spiral return gas tube.
Its function is to allow the gas to circulate within the discharge tube, facilitating constant gas exchange.
② Optical Resonator
The optical resonator is composed of a total reflection mirror and a partial reflection mirror, making up a crucial part of the CO2 laser.
The optical resonator typically has three functions: controlling the direction of the light beam propagation, enhancing monochromaticity; selecting a mode; extending the working length of the active medium.
The simplest and most commonly used laser’s optical resonator is composed of two flat mirrors (or spherical mirrors) placed opposite each other. The resonator of the CO2 laser often uses a flat-concave cavity, with the reflection mirror made from K8 optical glass or optical quartz, processed into a concave mirror with a large radius of curvature.
A highly reflective metal film—gold film—is deposited on the mirror surface, achieving a reflection rate of 98.8% for light with a wavelength of 10.6μm, and it has stable chemical properties.
We know that the light emitted by carbon dioxide is infrared, so the mirror needs to be able to transmit infrared light. Because common optical glass is opaque to infrared light, a small hole is required in the center of the total reflection mirror, which is then sealed with a material that can transmit a 10.6μm laser.
This seals the gas and allows a portion of the laser in the resonator to exit the cavity from this small hole, forming a laser beam.
③ Power Supply and Pump
The pump source provides energy to cause a population inversion between the upper and lower energy levels in the working material. The discharge current of a sealed CO2 laser is small, using a cold cathode, and the cathode is made into a cylindrical shape with molybdenum or nickel.
With a working current of 30-40mA and a cathode cylinder area of 500cm2, the mirror will not become contaminated. A light barrier is added between the cathode and the mirror.
As shown in Figure 2, the diagram illustrates the molecular energy levels responsible for laser generation in a CO2 laser.
The excitation process of the CO2 laser, as can be discerned from Figure 2, mainly involves three gases: CO2, nitrogen, and helium. CO2 is the gas that produces laser radiation, while nitrogen and helium serve as auxiliary gases.
The helium serves two purposes: it accelerates the thermal relaxation process of the 010 level, which aids in the extraction from the 100 and 020 levels and facilitates effective heat transfer.
The introduction of nitrogen primarily facilitates energy transfer in the CO2 laser, contributing significantly to the accumulation of particles at the upper energy levels of CO2 laser and the output of high-power, high-efficiency lasers.
The pump employs continuous DC power excitation. Its DC power principle involves transforming the connected AC voltage using a transformer, then rectifying and filtering the high voltage to apply it to the laser tube.
The CO2 laser is a high-efficiency laser that minimizes damage to the working medium. It emits an invisible laser with a wavelength of 10.6μm, making it an ideal laser.
According to the working condition of the gas, it can be divided into closed and circulation types. Based on the excitation method, it can be divided into electric excitation, chemical excitation, thermal excitation, optical excitation, and nuclear excitation. Nearly all CO2 lasers used in medicine are electrically excited.
The basic operating principle of the CO2 laser is similar to other molecular lasers, with the stimulated emission process being rather complex.
The molecule has three different movements: the electrons’ movement within the molecule, which determines the molecule’s electronic energy state; the atoms’ vibrations within the molecule, i.e., atoms oscillating periodically around their equilibrium positions, determining the vibrational energy state of the molecule; and the rotation of the molecule, i.e., the continuous rotation of the molecule in space as a whole, determining the rotational energy state of the molecule.
Molecular movements are extremely complex, hence the complexity of the energy levels.
Laser Generation in CO2 Laser: In the discharge tube, a DC current of several tens to hundreds of milliamperes is typically input.
During discharge, the nitrogen molecules in the mixed gas within the discharge tube are excited due to electron collision. The excited nitrogen molecules then collide with CO2 molecules.
The N2 molecule transfers its energy to the CO2 molecule, causing the CO2 molecule to transition from a lower energy level to a higher one, resulting in a population inversion and, consequently, laser generation.
Compared to other lasers, CO2 lasers have the following advantages and disadvantages:
Advantages:
They exhibit superior directionality, monochromaticity, and frequency stability. Given the low density of the gas, it’s difficult to achieve a high density of excited particles, so the energy density output of a CO2 gas laser is generally lower than that of a solid-state laser.
Disadvantages:
While the energy conversion efficiency of CO2 lasers is quite high, it will not exceed 40%. This means that over 60% of the energy is converted into thermal energy of the gas, resulting in an increase in temperature. The rise in gas temperature can cause depopulation of the upper laser level and thermal excitation of the lower level, both of which decrease the number of particle inversions.
Furthermore, an increase in gas temperature can cause spectral line broadening, leading to a decrease in the gain coefficient.
Especially, the rise in gas temperature may also cause decomposition of CO2 molecules, reducing the concentration of CO2 molecules in the discharge tube. These factors can decrease the output power of the laser, and even lead to “thermal quenching”.
Over recent years, the steady development of CO2 lasers has been notable in military applications. Laser weapons, as a new concept, have become favored in the weaponry of the new century due to their advantages over traditional conventional weapons, such as high speed, good directionality, high energy density, and high operational efficiency.
High-energy laser weapons are playing an increasingly important role in military applications, representing the direction of future weaponry development. They are poised to profoundly change the current battlefield environment and modes of warfare, deeply transforming the nature of future conflicts.
High-energy aerodynamic CO2 lasers with high output power have been designed by various countries for the development of high-energy laser weapons.
A basic feature of laser missile defense, or laser anti-missile tactics, is the use of high-energy lasers traveling at the speed of light to destroy missiles or other flying objects moving at the speed of sound.
We can confidently say that this area is dominated by CO2 lasers due to their significant advantages.
Currently, the army is adopting land-based small laser anti-missile systems, while the air force is using airborne laser anti-missile systems, and the navy is using shipborne laser anti-missile systems, all of which use high-energy CO2 lasers.
The primary characteristics of future CO2 laser weapons are ultra-high power and high portability. High-energy lasers will be a crucial component of future combat systems, contributing to counter-surveillance, active protection, air defense, and mine clearance.
The high portability will greatly enhance the combat capabilities of individual soldiers, maximizing the role of each soldier, although this idea is currently theoretical. Laser weapons of various countries are being developed in this direction.
Future CO2 laser weapons are expected to evolve towards high functionality, portability, and lethal efficiency. As shown in Figure 3:
Over the past 20 years, laser technology has rapidly advanced in the medical field, effectively curing many diseases and congenital disorders.
Free-beam CO2 lasers are used in surgeries, often in a non-contact manner with skin tissue, providing various advantages over conventional surgeries, such as reducing mechanical damage, increasing protection of surrounding tissues, and maintaining aseptic conditions more easily.
Compared to other laser surgeries, the CO2 laser scalpel has stronger cutting power, a higher tissue absorption coefficient, and a smaller tissue penetration concentration (approximately 0.23mm). This makes it less likely to damage arteries during surgery, leading to the widespread use of continuous CO2 lasers for clinical surgical treatment.
However, the damage of continuous CO2 lasers to tissues in clinical applications is non-selective, often resulting in side effects like skin scars after surgery. The cutting or vaporization of lesions can also harm normal tissues to varying degrees, making it unsuitable for surgeries with high requirements. This significantly limits the further application of CO2 lasers in medicine.
In 1983, Aderson and Parrish proposed the principle of “selective photothermolysis” for non-damaging laser treatment.
The essential idea is that when the laser passes through normal tissue to reach the target lesion, the lesion’s absorption coefficient for the laser should be higher than that of the normal tissue—the greater the difference, the better—to avoid damaging normal tissue when destroying the target lesion.
The thermal relaxation time of the target tissue should be longer than the pulse width or action time of the laser, preventing the heat from spreading to the surrounding normal tissue during the laser heating process.
Based on the principle of “selective photothermolysis,” high-energy pulse medical devices represented by ultra-pulse CO2 laser treatment machines emerged in the 1990s.
These devices have been successfully applied, allowing for breakthrough progress in high-requirement applications, especially dominating in the field of laser cosmetics. The prospects for development are very broad.
Ultra-pulse CO2 lasers employ advanced pulse technology and PWM power control technology. These not only quickly increase the peak power output of the laser, providing sufficient energy to the target tissue, but also precisely control the width and repetition frequency of each pulse through PWM signals.
By calculating the thermal relaxation time of the target tissue, controlling the pulse width can achieve optimal surgical results. For instance, the thermal relaxation time of capillaries is about 10μs, requiring a pulse width smaller than 10μs; the thermal relaxation time of skin tissue is approximately 1 ms, necessitating a pulse width smaller than 1 ms for a laser device used for skin resurfacing and wrinkle removal.
The most significant difference between modern laser devices and those from over a decade ago lies in the precise control of pulse width, which fundamentally ensures the safety of modern laser treatment.
Ultra-pulse CO2 laser treatment machines not only share the common characteristics of continuous CO2 laser scalpels but also have their advantages. They can output high-energy, high-repetition-frequency pulsed lasers, meeting the operational requirements of “laser selective photothermolysis.”
They can quickly and effectively remove target lesion tissues, minimizing laser damage to normal tissues, and significantly increasing the accuracy and safety of medical clinics.
Clinical practice has shown that when performing the same surgery, the laser power used by pulsed lasers is much lower than that of continuous lasers.
Therefore, the tissue reaction caused by laser surgery is lighter, the damage to surrounding tissues is less, the time is shorter, and less smoke is produced during treatment, giving a clear visual field.
Ultra-pulse CO2 lasers have been widely used in Otorhinolaryngology, Gynecology, Neurosurgery, General Surgery, and Aesthetics.
Lumenis, the company that introduced Bridge Therapy, has researched and produced various CO2 laser treatment devices, such as the NovaPulse Series for use in Otorhinolaryngology and Aesthetics.
Other examples include the MODEL CTL1401 surgical device produced by Poland’s CTL company, and Japan’s NANO LASER’s GL-Ⅲ, a CO2 laser treatment device for oral surgery.
(1) CO2 Laser Cutting Technology
Laser cutting technology is extensively used in the processing of both metallic and non-metallic materials. It significantly reduces processing time, lowers costs, and improves the quality of workpieces.
Laser cutting is achieved by the high-power density energy produced after laser focusing.
Compared to traditional sheet metal processing methods, laser cutting offers superior cutting quality, speed, flexibility (permitting arbitrary shapes), and broad material adaptability.
In terms of metal cutting, it constitutes the primary domain of CO2 laser cutting. Currently, considering economic factors, high-power laser cutting machines are generally employed for subcontracting in station processing format.
With the maturation of medium-power CO2 lasers domestically, various sheet metal factories will purchase their own laser cutting machines, leading to a substantial increase in demand.
Non-metal cutting is applied in die template cutting, wood and high-density fiberboard cutting, and plastic cutting.
(2) CO2 Laser Welding Technology
Laser welding is a material joining method, predominantly used for the connection of metal materials. Similar to traditional welding techniques, it connects two components or parts by melting the material in the connection area.
Given the high concentration of laser energy, the heating and cooling processes are incredibly swift.
Materials that are challenging to process with standard welding techniques, due to their brittleness, high hardness, or strong flexibility, can be easily managed with lasers.
On the other hand, laser welding involves no mechanical contact, making it easy to ensure that the welding area does not deform under stress.
By melting the smallest quantity of material to achieve alloy connections, the quality of welding is greatly enhanced, and productivity is increased.
Laser welding offers a deep weld seam and minimal heat-affected zone, resulting in superior quality.
For instance, in the welding of metal thin plates, medium-power CO2 lasers are suitable for welding metal thin plates with a thickness of less than 1mm, such as laminated silicon steel sheets often used in automobile parts, generators, wipers, starters, window lifters, etc.
These were previously secured by punching and riveting, but now can be welded by lasers.
Battery welding, particularly in the production of lithium batteries – like tab welding, safety valve welding, negative electrode welding, shell sealing welding – laser welding is the optimal process, requiring a large variety and number of laser welding machines.
The demand for laser welding in precision instrument parts is also increasing, such as the welding of stainless steel diaphragms and aviation instrument casings.
For the nearly 50 years since its inception, the CO2 laser has been the focus of human attention. This type of gas laser operates using CO2 gas as its working medium. CO2 lasers are a significant category of gas lasers.
The current main research directions for CO2 lasers include:
1. High-efficiency CO2 lasers.
Undoubtedly, compared to solid-state lasers, their efficiency is extremely high. However, overall, relative to the CO2 laser itself, the efficiency is still comparatively low.
In 1964, by employing N2, a conversion efficiency of 3% was achieved; in 1965, by using a mixture of CO2-N2-He gases, the conversion efficiency reached 6%. To date, the highest efficiency does not exceed 60%.
Many companies are researching efficiency improvements. For example, the American company Datong has achieved an efficiency of about 60% in their CO2 lasers.
2. Small, multi-functional CO2 lasers.
Most of the present CO2 lasers have a single function and can only carry out a very specific task. We know that the CO2 lasers used in large hospitals for removing freckles and hair are quite bulky, but their structures are fundamentally the same. Using multi-functional CO2 lasers results in smaller physical volume and, relatively, a much lower price.
3. High-power CO2 lasers.
High power has always been a military pursuit. In this respect, the research level of some domestic military enterprises is relatively backward. The U.S. Air Force was the first to start researching high-power CO2 lasers.
In 1975, the eleventh anniversary of the birth of the CO2 laser, the U.S. Air Force developed a CO2 laser with a power level reaching 30KW. In 1988, the output power of the researched CO2 laser reached 380KW.
According to some data released by the U.S. military, the output power of the developed CO2 lasers has now reached the level of tens of megawatts.
4. Research on industrial technology.
CO2 lasers dominate in laser processing, being widely used for welding, cutting, heat treatment, and cleaning, among other things. The quality and power output of the laser have very precise requirements.
Therefore, industrial CO2 lasers need to have high-quality laser beams and stable output power.
Laser applications have already permeated fields such as optics, medicine, nuclear energy, astronomy, geography, and oceanography, marking the development of the new technological revolution.
If you compare the history of laser development with the history of electronics and aviation, you must realize that we are still in the early stages of laser development, and an even more exciting and promising future is on the horizon.
The future of CO2 lasers will evolve in the following directions:
(1) High-Power Transverse-Flow CO2 Laser.
This high-power transverse-flow CO2 laser is used for laser processing and heat treatment, with an integrated box-shaped structure. The upper box of the unit houses an integrated discharge chamber, heat exchanger, fan system, inlet/outlet guide, and an optical resonator.
The lower box contains the laser power source, gas charging and discharging system, vacuum pump, ballast resistor box, and control box.
Compared to existing technology, it features a compact structure, easy installation, maintenance, high working efficiency, and can be miniaturized.
Its primary applications are in the welding of diamond tools, automotive gears, automotive airbag gas generators, laser surface hardening and overlaying processes, and unique applications like surface repair of petrochemical parts and surface fusion hardening of steel rolls.
(2) Acousto-Optic Q-Switched CO2 Laser.
To meet the application requirements in fields like laser ranging, environmental detection, space communication, and the research of laser-material interaction mechanisms, an acousto-optic Q-switched CO2 laser has been developed.
By using the rate equations of Q-switched pulse lasers, the main technical parameters of the laser output have been analyzed theoretically, calculated, and then experimentally verified.
The pulse repetition frequency of the laser is from 1Hz to 50 kHz. At 1 kHz operation, the output laser pulse width is 180 ns, and the peak power is 4062 W, which is basically consistent with theoretical calculations.
The results show that a high repetition frequency, narrow pulse width, high peak power output of a small-sized CO2 laser can be achieved by optimal selection of the acousto-optic (AO) crystal and reasonable resonator design.
Wavelength tuning and coded output of such lasers can be achieved through grating line selection design and TTL signal control.
(3) Compact Long-Lifetime RF Excited Waveguide CO2 Laser.
To broaden the application of CO2 lasers in industrial processing and military use, a compact long-lifetime RF excited waveguide CO2 laser has been developed by using aluminum alloy extruded profiles for the laser body, disk inductance instead of traditional wire-wound inductance, and an all-metal sealing process.
It can output continuously or pulse output at a modulation frequency not higher than 20kHz, with a maximum output power of 30W, a working life exceeding 1500 hours, and a storage life exceeding 1.5 years.
The results show that this laser features a compact structure, stable output power, long working life, and can work in continuous and pulse modulation modes. It not only can process various materials but also be used in military applications.
(4) New Portable TEA CO2 Laser.
This is a new portable transversely excited atmospheric pressure CO2 laser. The laser is powered by four No. 5 rechargeable batteries and can operate continuously for 1 hour at a repetition rate of 1 Hz.
The size of the complete laser unit (including the power supply and control system) is 200 nm×200 mm × 360 mm, and its weight is less than 8 kg. The laser uses ultraviolet corona preionization for stable and uniform discharge.
In free oscillation conditions, the laser pulse output energy reaches 35 mJ, and the output pulse width is 70 ns.
(5) High-Power Continuous CO2 Laser.
In response to the issue of cracks and blade deformation in the continuous laser cladding of helicopter engine turbine blades, a new power control scheme was adopted on a 5 kW continuous transverse-flow CO2 laser.
Through software and related controls, pulsed laser power output was achieved, overcoming the cost and stability problems brought about by high-power switching power supplies.
The pulse modulation frequency can reach 5 Hz, and the modulation duty cycle can range from 5% to 100%.
In an experiment of alloy powder Stellite X-40 cladding on the K403 alloy surface of engine blades, a peak power of 4 kW, pulse repetition frequency of 4 Hz, and duty cycle of 20% were used.
The results showed that the heat-affected zone was reduced by 50% after cladding, hardness increased by 5%, the interfacial bonding performance was comparable to the base material, and there were no cladding cracks or blade deformation.