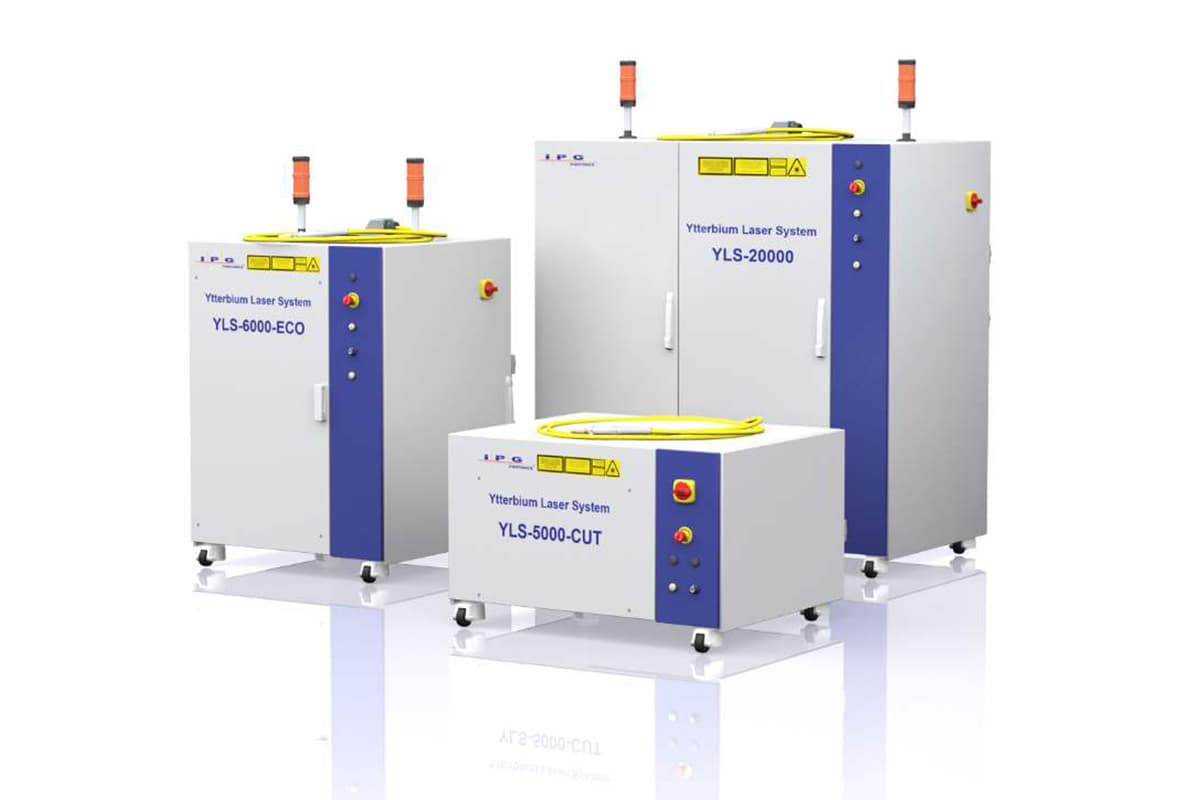
Have you ever wondered how fiber laser technology has revolutionized various industries? This article explores the core principles and diverse applications of fiber lasers, highlighting their advantages over traditional laser systems. Discover how these compact, high-precision tools are used in fields ranging from telecommunications to medical technology, offering superior beam quality and energy efficiency. By reading, you’ll gain insights into the mechanisms that make fiber lasers a vital part of modern engineering and industry advancements.
Research on doped fiber lasers utilizing gain media dates back to the 1960s when Snitzer reported in 1963 the creation of a fiber laser with neodymium ions (Nd3+) doped in a glass matrix.
Since the 1970s, significant progress has been made in fiber preparation technology and the exploration of pump and resonant cavity structures for fiber lasers.
In the mid-1980s, a breakthrough in doped fiber (Er3+) at the University of Southampton in the UK greatly enhanced the practicality of fiber lasers, showing very promising application prospects.
Compared with traditional solid and gas lasers, fiber lasers have many unique advantages, such as high beam quality, small size, lightweight, maintenance-free, air-cooled, easy to operate, low operating cost, and long-term use in industrial environments.
They also offer high processing precision, fast speed, long life, energy saving, and excellent flexibility for intelligence and automation. Therefore, they have replaced traditional YAG and CO2 lasers in many fields.
The output wavelength range of fiber lasers is between 400-3400nm, applicable in various fields such as optical data storage, optical communication, sensor technology, spectroscopy, and medical applications.
Currently, rapid development is seen in doped fiber lasers, fiber Bragg grating lasers, tunable narrow linewidth fiber lasers, and high-power double-clad fiber lasers.
The fiber laser primarily consists of three parts: the gain medium that can generate photons, the optical resonant cavity that enables photon feedback and resonant amplification in the gain medium, and the pump source that can excite the laser medium.
The basic structure of the fiber laser is shown in Figure 2.1.
The gain medium is a fiber core doped with rare earth ions. The doped fiber is placed between two mirrors with selected reflectivity. The pump light is coupled into the fiber from the left mirror of the fiber laser and outputs laser light through a collimating optical system and a filter.
Theoretically, the pump source and the gain fiber are the essential components of the fiber laser, and the resonant cavity is not indispensable. The mode selection of the resonant cavity and the lengthening of the gain medium are not necessary in fiber lasers because the fiber itself can be very long, thereby obtaining a very high single-pass gain, and the waveguide effect of the fiber can play a mode selection role.
However, in practical applications, people generally prefer to use shorter fibers, so in most cases, a resonant cavity is used to introduce feedback.
Due to the waveguide structure of fiber lasers, they can accommodate strong pumping and have a high gain (single-pass gain up to 50dB). Rare earth elements in the glass matrix have a wide linewidth and tuning range (Yb3+ is 125nm, Tm3+ >300nm).
The specific features are as follows:
1) The fiber serves as a waveguide medium, offering high coupling efficiency, small core diameter, and ease in forming high power density within the fiber. It can conveniently connect with current fiber optic communication systems. The resulting lasers have high conversion efficiency, low laser threshold, excellent beam quality, and narrow linewidth.
2) Given the high “surface area/volume” ratio of the fiber, it has good heat dissipation. The environmental temperature can range from -20 to 70℃, eliminating the need for a large water cooling system and only requiring simple air cooling.
3) The fiber laser can operate under harsh conditions, such as high impact, high vibration, high temperature, and dusty conditions.
4) Due to the excellent flexibility of the fiber, the laser can be designed to be quite small and flexible, with a compact shape and small volume, making it easy for system integration and offering a high performance-price ratio.
5) The fiber laser has quite a lot of tunable parameters and selectivity, enabling it to cover a wide tuning range, excellent monochromaticity, and high stability. It has a long pump life, with an average fault-free working time of 10kh or even over 100kh.
The currently developed fiber lasers mainly use fibers doped with rare earth elements as the gain medium.
The working principle of the fiber laser is that the pump light is incident on the doped fiber through the front reflector (or front grating), and the rare earth ions that have absorbed the photon energy will undergo energy level transitions, achieving “particle number inversion”.
The inverted particles will transition back to the ground state in the form of radiation after relaxation, simultaneously releasing energy in the form of photons, and outputting the laser through the rear reflector (rear grating).
The fiber amplifier doped with rare earth elements has promoted the development of fiber lasers, because fiber amplifiers can form fiber lasers through appropriate feedback mechanisms.
When the pump light passes through the rare earth ions in the fiber, it will be absorbed by the rare earth ions. At this time, the rare earth atoms that absorb photon energy will be excited to a higher lasing energy level, thereby achieving ion number inversion.
The inverted ion number will transition from the high energy level to the ground state in the form of radiation, and release energy, completing the stimulated radiation. The radiation mode from the excited state to the ground state has two types: spontaneous radiation and stimulated radiation.
Among them, stimulated radiation is a radiation of the same frequency and phase, which can form a very coherent laser. Laser emission is a physical process where stimulated radiation far exceeds spontaneous radiation.
In order for this process to continue, ion number inversion must be formed. Therefore, the energy levels involved in the process should exceed two, and there must also be a pump source to provide energy.
The fiber laser can actually be called a wavelength converter, through which the pump wavelength light can be converted into the required lasing wavelength light.
For example, an erbium-doped fiber laser pumps 980nm light and outputs 1550nm laser. The output of the laser can be continuous or pulsed.
Fiber lasers have two lasing states, three-level and four-level lasing. The laser principles of the three-level and four-level are shown in Figure 2.2.
The pump (short-wavelength high-energy photon) causes the electron to transition from the ground state to the high energy state E44 or E33, then transitions to the upper laser level E43 or E32 through non-radiative transitions.
When the electron further transitions from the upper laser level to the lower energy level E42 or E31, the laser process will occur.
There are various types of fiber optic lasers which can be divided into different categories as shown in Table 3.1. The following sections will provide an introduction to several types of these lasers.
Table 3.1 Classification of Fiber Optic Lasers
Classification by Resonator Structure | F-P Cavity, Ring Cavity, Loop Reflector Fiber Resonator and “8” Shape Cavity, DBR Fiber Laser, DFB Fiber Laser |
Classification by Fiber Structure | Single Cladding Fiber Laser, Double Cladding Fiber Laser |
Classification by Gain Medium | Rare-Earth-Doped Fiber Laser, Nonlinear Effect Fiber Laser, Single Crystal Fiber Laser, Plastic Fiber Laser |
Classification by Working Mechanism | Up-Conversion Fiber Laser, Down-Conversion Fiber Laser |
Classification by Doping Elements | Erbium (Er3+), Neodymium (Nd3+), Praseodymium (Pr3+), Thulium (Tm3+), Ytterbium (Yb3+), Holmium (Ho3+) and 15 other types |
Classification by Output Wavelength | S-Band (1280-1350nm), C-Band (1528-1565nm), L-Band (1561-1620nm) |
Classification by Output Laser | Pulsed Laser, Continuous Wave Laser |
Rare earth elements encompass 15 elements, positioned in the fifth row of the periodic table.
Currently, the maturely developed rare earth ions incorporated in active fibers include Er3+, Nd3+, Pr3+, Tm3+, and Yb3+.
In recent years, double-clad doped fiber lasers, utilizing cladding pumping technology, have significantly increased the output power, becoming another research hotspot in the field of lasers.
This type of fiber structure, as shown in Figure 3.1, is composed of an outer cladding, inner cladding, and a doped core.
The refractive index of the outer cladding is less than that of the inner cladding, which in turn is less than the refractive index of the fiber core, thus forming a double-layer waveguide structure.
The doped double-clad fiber is a key component in the construction of fiber lasers. Its main roles in a fiber laser include:
1) Converting the pump light power into the working medium of the laser;
2) Collaborating with other devices to form a laser resonator.
Its working principle mainly involves injecting the pump light into the fiber either laterally or from the end face. Because the refractive index of the outer cladding is much lower than that of the fiber’s inner cladding, the inner cladding can transmit multimode pump light.
The cross-sectional dimension of the inner cladding is larger than the core. Thus, for the generated laser wavelength, the inner cladding and the rare earth doped core form a perfect single-mode waveguide, while it and the outer cladding form a multimode waveguide for transmitting pump light power.
This allows for large power multimode pump light to be coupled into the inner cladding. The multimode pump light is absorbed multiple times as it travels along the fiber, crossing the core. Due to the excitation of rare earth ions in the core, a high power signal laser output is produced.
The working principle is illustrated in Figure 3.1.
The growing maturity of UV-written fiber Bragg grating technology in the 1990s has led to increased attention on fiber Bragg grating lasers, primarily Distributed Bragg Reflector (DBR) and Distributed Feedback (DFB) fiber grating lasers.
The main difference between the two is that the DFB fiber laser uses only one grating to achieve optical feedback and wavelength selection, thus offering better stability and avoiding the fusion loss between Er-doped fiber and the grating.
However, while the grating can be directly written into the Er-doped fiber using UV, practical fabrication of the DEB fiber laser is not easy due to the low Ge content in the fiber core and poor photosensitivity.
In contrast, the DBR fiber laser can be fabricated more easily by fusing a Ge-doped fiber grating at both ends of the Er-doped fiber to form a resonant cavity.
DBR and DFB fiber grating lasers face several issues such as low pump absorption efficiency due to short resonant cavities, broader spectral lines than ring lasers, and mode hopping.
Continuous efforts are being made to solve these problems. Proposed improvements include using Er:Yb co-doped fiber as a gain medium, adopting an intracavity pumping method, and integrating the oscillator and power amplifier.
Ultra-short pulse lasers are currently a hot research topic in fiber lasers, primarily utilizing passive mode-locking techniques.
Similar to solid-state lasers, fiber lasers generate short pulse laser outputs based on the principle of mode-locking. When a fiber laser operates on a large number of longitudinal modes within the gain bandwidth, mode-locking is achieved when each longitudinal mode phase synchronizes and the phase difference between any two adjacent longitudinal modes is constant.
The single pulse circulating in the resonant cavity outputs energy through the output coupler. Fiber lasers are divided into active mode-locked fiber lasers and passive mode-locked fiber lasers.
The active mode-locking modulation capability limits the pulse width of the mode-locked pulse, which is generally on the order of picoseconds. Passive mode-locked fiber lasers utilize the nonlinear optical effects of the fiber or other optical components to achieve mode-locking.
The laser structure is simple and can achieve self-starting mode-locking under certain conditions without any modulation components. Using passive mode-locked fiber lasers can output ultra-short pulses on the order of femtoseconds.
Ultra-short pulse lasers have been used in ultra-fast light sources, resulting in a variety of time-resolved spectroscopy and pumping techniques. Ultra-short pulse generation technology is key to achieving ultra-high-speed optical time division multiplexing (OTDM). Ultra-short pulse fiber lasers are widespread across various fields such as materials, biology, medicine, chemistry, and military.
Lasers are the core of laser technology, and the future development direction of fiber lasers will be to further improve the performance of fiber lasers, such as further increasing output power and improving beam quality; expanding new laser wavelengths, expanding the tunable range of lasers; narrowing the laser spectrum; developing ultra-short pulses (ps and fs levels) of high-brightness lasers; and carrying out research on overall miniaturization, practicality, and intelligence.
In recent years, development has mainly focused on three aspects:
(1) improving the performance of fiber Bragg gratings, allowing them to be well applied in fiber lasers;
(2) fiber lasers with narrower pulse and spectral line widths, higher output power, wider tuning range, etc.;
(3) making fiber lasers more practical.
Industrial Applications: The most notable application of fiber lasers in industry is material processing. With its continually increasing power, fiber lasers have started to be used on a large scale for industrial cutting.
Fiber lasers are ideal for cutting, processing, and handling both metallic and non-metallic materials. They can be used for laser product calibration, precision cutting, laser engraving, laser welding, precision drilling, laser detection, micro bending, laser measurement, and other technical aspects.
Telecommunication Applications: To meet the current requirements for high-capacity communication, the application of fiber lasers has become an emerging technology in communication.
Future communication technology will gradually transition from electrical to optical communication. Fiber lasers can not only generate continuous laser output but also produce ultra-short laser pulses of picoseconds (ps) or even femtoseconds (fs).
Fiber lasers have made great strides in reducing thresholds, broadening wavelength ranges, and tunable wavelength capabilities. Soliton communication, a practical technology, can reach a transmission distance of millions of kilometers, a transmission rate of 20Gb/s, and a bit error rate lower than 10-13, achieving high-speed and high-quality signal transmission.
Military Applications: With the continuous increase in the power of fiber lasers, their application in the military is becoming more and more widespread.
To achieve the purpose of directed energy weapons, several fiber lasers are combined into a coherent array structure, which can increase the power of fiber lasers.
At the Air Force Research Laboratory in the United States, research is currently being conducted on 100kW fiber lasers to meet military application objectives.