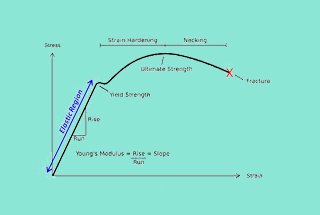
Imagine your car engine failing in the middle of a scorching desert because the materials couldn’t withstand the heat. This scenario underscores the vital importance of understanding how high temperatures impact the mechanical properties of materials. In this article, we’ll explore how prolonged exposure to high temperatures affects metal structures, causing phenomena like diffusion and recrystallization, and what engineers can do to mitigate these effects. Dive in to learn how temperature and time shape material performance, ensuring safety and reliability in high-temperature environments.
The structures of metals and alloys change due to phenomena such as diffusion, recovery, recrystallization and others at high temperatures.
Additionally, prolonged exposure to high temperatures can harm the performance of metal materials.
In equipment such as high-pressure steam boilers, steam turbines, diesel engines, aeroengines, chemical equipment, and high temperature and high pressure pipelines, many parts are in service under high temperatures for extended periods.
It is not enough to only consider the mechanical properties of such materials under normal temperature and short-term static load. For instance, high temperature and high pressure pipes in chemical equipment, although the stress they bear is lower than the yield strength of materials at their working temperature, will undergo continuous plastic deformation over time, which gradually increases the pipe diameter and can even lead to pipe rupture.
The classification of “high” or “low” temperature is relative to the melting point of the metal. The ratio of temperature to melting point (T/Tm) is often used as a reference, where Tm refers to the melting point of the material. If T/Tm is greater than 0.4 to 0.5, it is considered as high temperature.
The temperature of a civil aircraft is approximately 1500°C, while that of a military aircraft is around 2000°C. The local working temperature of spacecraft can even reach 2500°C.
Temperature has a significant impact on the mechanical properties of materials. The duration of loading at high temperatures also has a major influence on these properties. It is important to note that high-temperature mechanical properties are not the same as room temperature mechanical properties.
As a general trend, as temperature increases, the strength of metal materials decreases while their plasticity increases. The duration of the load also affects the mechanical properties. Under short-term loads, the tensile strength decreases and plasticity increases, but over long-term loads, plasticity decreases significantly, notch sensitivity increases, and brittle fracture often occurs.
The combined effect of temperature and time also influences the fracture path of the material. For instance, creep may occur during long-term use, eventually leading to fracture. The tensile strength of steel at high temperature decreases with the extension of the load duration.
As temperature increases, both grain strength and grain boundary strength decrease. However, grain boundary strength decreases more rapidly due to the irregular arrangement of atoms at the grain boundary, making it easier for diffusion to occur.
The temperature at which grain strength and grain boundary strength are equal is known as the “equal strength temperature” (TE). When the material operates above TE, the fracture mode of the material changes from the typical transgranular fracture to intergranular fracture.
It’s important to note that TE is not fixed and is influenced by the deformation rate. Since grain boundary strength is more sensitive to deformation rate than grain strength, TE increases with the increase of the deformation rate.
In conclusion, to study the mechanical properties of materials at high temperatures, both temperature and time must be considered as factors.
Creep is the gradual plastic deformation of metal that occurs under constant temperature and load, even if the stress is lower than the yield strength at that temperature, over an extended period of time. This type of material fracture caused by creep deformation is referred to as creep fracture.
Although creep can occur at low temperatures, it is only significant when the temperature is greater than approximately 0.3. If the temperature of carbon steel exceeds 300°C or that of alloy steel exceeds 400°C, the effect of creep must be taken into account.
It’s important to note that the creep curve of the same material varies with stress and temperature.
Typical creep curve
The first stage, labeled as “ab,” is known as the Deceleration Creep Stage or the Transition Creep Stage. The creep rate at the start of this stage is very high and gradually decreases over time until it reaches its minimum at point “b.”
The second stage, labeled as “bc,” is referred to as the Constant Speed Creep Stage or the Steady State Creep Stage. This stage is characterized by a relatively constant creep rate. The creep rate of a metal is usually expressed by the creep rate ε during this stage.
The third stage is the Accelerated Creep Stage. As time progresses, the creep rate gradually increases until creep fracture occurs at point “d.”
Change diagram of creep curve with different stress and temperature
As depicted in the figure, when the stress is low or the temperature is low, the second stage of creep lasts for a considerable amount of time, and in some cases, the third stage may not even occur. On the other hand, when the stress is high or the temperature is high, the second stage of creep is very brief or may not happen at all, resulting in the specimen breaking down in a very short time.
Macro characteristics of the fracture surface
Plastic deformation occurs close to the fracture surface and there are numerous cracks in the vicinity of the deformed area (these cracks can be seen on the surface of the fractured part). In instances of high-temperature oxidation, the fracture surface is coated with a layer of oxide film.
Micro characteristics of the fracture surface
Intergranular fracture morphology of crystal sugar like patterns
The creep limit, rupture strength, relaxation stability, and other mechanical properties are commonly used to evaluate the creep behavior of materials.
The creep limit is a measure of a metal material’s resistance to plastic deformation under long-term loading at high temperatures and is a crucial factor for the selection and design of high-temperature service components.
There are two ways to express the creep limit in MPa: one is to determine the maximum stress that the specimen can endure at a specified steady creep rate within a specified time and temperature; the other is to determine the maximum stress that causes the specimen to undergo a specified creep elongation within a specified time and temperature.
Example 1 shows that the creep limit of the material is 80MPa when the temperature is 500 ℃ and the steady creep rate is 1×10-5%/h;
Example 2 shows that the creep limit of the material is 100MPa when the temperature is 500 ℃, 100000 hours, and the creep elongation is 1%.
Creep test equipment and schematic diagram
The creep test must be performed under consistent temperature conditions and a variety of stress levels, with a minimum of 4 creep curves recorded.
The creep curves should be created based on the recorded results, with the slope of the straight line on the curve representing the creep rate.
The relationship curve is plotted on logarithmic coordinates using the obtained stress creep rate data.
By applying relatively high stress levels, multiple creep curves can be generated with relatively short testing times. The stress value for a specified creep rate can be determined through interpolation or extrapolation of the measured creep rate, allowing for the determination of the creep limit.
At a constant temperature, there is a linear empirical relationship between the second-stage creep stress (σ) and the steady creep rate (ε) in double logarithmic coordinates.
S-590 alloy σ- ε curve
(20.0%Cr, 19.4 %Ni, 19.3%Co, 4.0%W, 4.0%Nb, 3.8%Mo, 1.35%Mn, 0.43%C)
Durable strength refers to a material’s ability to resist fracture over a long period of time under high temperature loads. It is the maximum stress that a material can withstand without undergoing creep fracture under specific temperature and time conditions. Durable strength is a measure of a material’s fracture resistance, while creep limit refers to its resistance to deformation.
For some materials and components, creep deformation is minimal and their only requirement is to not break during their service life (such as the superheated steam pipe in a boiler). In these cases, endurance strength is the primary criterion used to evaluate the suitability of the material or component for use.
Stress rupture strength curve of S-590 alloy
The endurance strength of metal materials is determined by high temperature tensile endurance test.
During the testing process, measuring the sample’s elongation is not necessary as long as the time it takes to fracture under a specified temperature and stress level is recorded.
For machine components with long design lives (tens of thousands to hundreds of thousands of hours or more), it is challenging to perform long-term tests, so the data is typically generated using high stress levels and short fracture times. The endurance strength of the materials is then calculated through extrapolation.
Extrapolate empirical formula:
(t-fracture time, σ-stress, A, B-constants related to test temperature and material)
Take the logarithm of the above formula to get:
Make log t-log σ Fig., the linear relationship can be extrapolated from the data with short fracture time to the lasting strength with long time.
When subjected to constant deformation, the elastic stress of materials gradually decreases over time, known as stress relaxation.
The resistance of metal materials to stress relaxation is referred to as relaxation stability, which can be determined through stress relaxation testing by measuring the stress relaxation curve.
Residual stress is a metric used to evaluate the relaxation stability of metal materials. The higher the residual stress, the better the relaxation temperature.
Stress relaxation curve
Stage 1: the stress drops rapidly at the beginning;
Sstage 2: the stage where the stress drop gradually slows down;
Relaxation limit: under certain initial stress and temperature, the residual stress will not continue to relax.
In order to enhance the creep limit, it is important to control the rate of dislocation climb based on the creep deformation and fracture mechanism.
To improve the rupture strength, controlling grain boundary sliding and vacancy diffusion is necessary.
Several factors can impact high-temperature mechanical properties, including chemical composition, the smelting process, heat treatment process, and grain size.
Influence of chemical composition of the alloy
The base materials for heat-resistant steels and alloys typically consist of metals and alloys with high melting points, high self-diffusion activation energy, or low stacking fault energy.
Metals with higher melting points, such as Chromium (Cr), Tungsten (W), Molybdenum (Mo), and Niobium (Nb), have slower self-diffusion rates.
Low stacking fault energy makes it easier to form extended dislocations and harder for dislocations to cross-slip and climb.
The dispersed phase can effectively block dislocation slip and climb.
Adding elements such as boron and rare earths, which increase the activation energy of grain boundary diffusion, not only hinder grain boundary sliding but also raise the surface energy of grain boundary cracks.
Heat-resistant materials with face-centered cubic structures have higher high-temperature strength compared to those with body-centered cubic structures.
Influence of smelting process
Revised:
Reducing the content of inclusions and metallurgical defects is important.
By using directional solidification, the number of transverse grain boundaries is reduced, leading to an improvement in rupture strength as cracks are more likely to form on transverse grain boundaries.
Influence of heat treatment process
Pearlitic heat-resistant steel typically undergoes a normalizing process followed by high temperature tempering.
The tempering temperature should be 100 to 150 degrees Celsius higher than the service temperature to enhance the structural stability under operating conditions.
Austenitic heat-resistant steel or alloys are typically treated through solution and aging to attain appropriate grain size and improve the distribution of strengthening phases.
Thermomechanical treatment can further enhance the strength of the alloy by altering the shape of grain boundaries (forming serrations) and creating polygonal subgrain boundaries within the grain.
Effect of grain size
Grain size: When the operating temperature is below the constant strength temperature, fine-grain steel exhibits higher strength, while when the operating temperature exceeds the constant strength temperature, coarse-grain steel possesses greater creep resistance and endurance strength.
Uneven grain size: When stress concentrates at the junction between large and small grains, cracks are more likely to form and result in premature fracture.