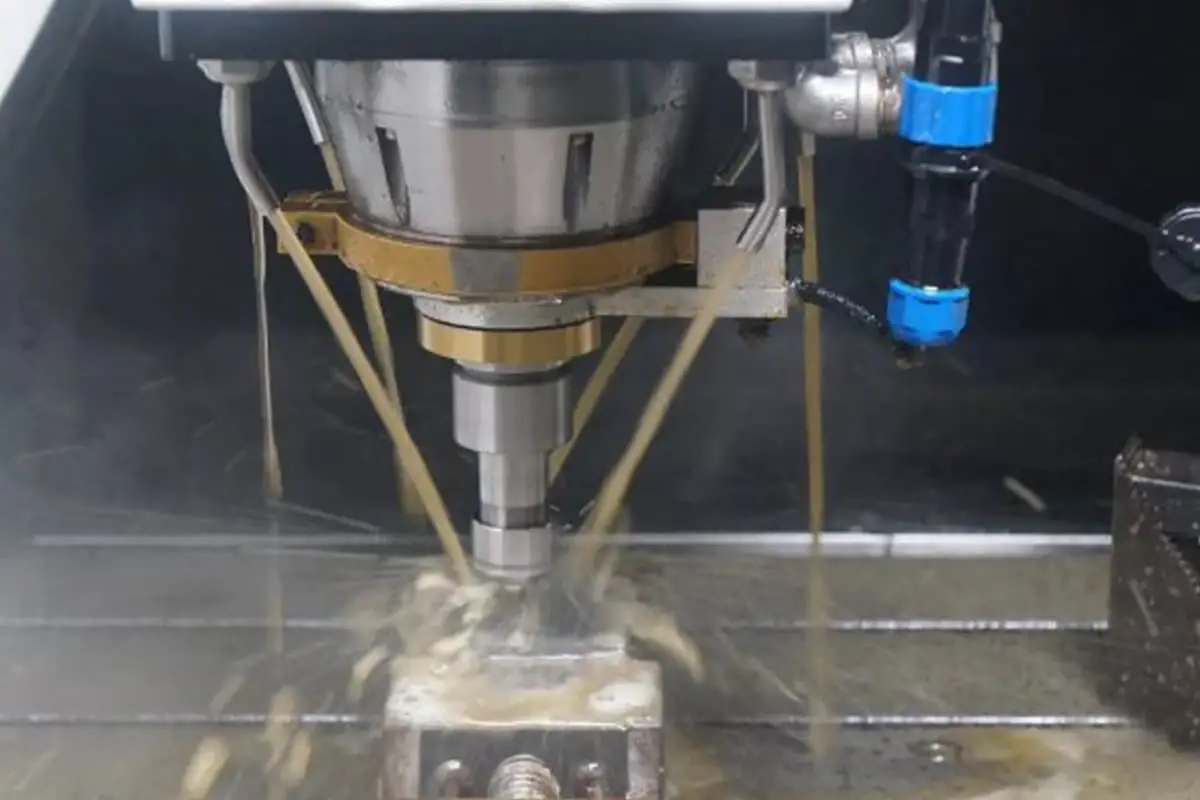
Imagine a technology that can effortlessly machine the toughest materials with precision and efficiency. Ultrasonic machining does just that, revolutionizing how industries handle hard, brittle, and composite materials. By harnessing ultrasonic vibration energy, this technique minimizes tool wear and enhances surface quality, making it indispensable in aerospace, automotive, and medical fields. Dive into this article to explore the principles, applications, and future trends of ultrasonic machining technology, and discover how it’s set to transform modern manufacturing.
This article provides an overview of the development, current research status, and future trends of ultrasonic machining technology, with a focus on its industrial applications.
Ultrasonic machining is a specialized technology used for machining difficult-to-process materials such as hard brittle materials, composite materials, and challenging metal materials. It has significant potential for use in various industries, including aerospace, automotive, semiconductor, 3C, and medical.
The technology works by using ultrasonic vibration energy to precisely remove material from difficult-to-machine materials.
Over the past few years, ultrasonic machining technology has seen significant development due to the collaboration of universities, research institutes, and companies both domestically and internationally. There have been numerous successful applications of ultrasonic machining in precision machining of various challenging materials.
As a representative of advanced manufacturing technology, ultrasonic machining will play a crucial role in improving the overall level of manufacturing.
In recent years, advanced engineering materials such as titanium alloys, superalloys, engineering ceramics, ceramic matrix composites, and honeycomb composites have emerged in various manufacturing fields including aerospace, automotive, semiconductor, 3C, and medical. These materials have excellent performance but poor machinability and are considered difficult-to-machine materials.
Traditional mechanical manufacturing technology faces challenges when it comes to precision machining these materials. To overcome these limitations, ultrasonic machining (UM) has gained attention and become widely used.
Ultrasonic machining is a process technology that utilizes ultrasonic vibration energy to precisely remove material from difficult-to-machine materials. This technology concentrates the ultrasonic vibration energy on the tool’s working area through a series of structural transmission and transformation, resulting in an impact removal effect on the material being cut and improving its machinability.
Ultrasonic machining technology has several advantages in the machining process, such as reducing cutting force and heat, minimizing tool wear and edge collapse burrs, optimizing chip morphology, improving surface quality, reducing sub-surface damage, and enhancing machining efficiency.
It is important to note that ultrasonic machining technology is based on power ultrasonic technology and is a physical removal process that does not alter material properties.
Due to the increasing market demand, commercial standardized systems in ultrasonic machining technology have become a focus of the current market. Relevant ultrasonic machining technologies have been applied in precision machining of various difficult-to-machine materials such as optical glass, sapphire, ceramics, alumina ceramics, titanium alloys, superalloys, carbon fiber composites, and aluminum-based silicon carbide composites.
Many scientific research institutions and manufacturing enterprises are now applying ultrasonic machining technology in the industry.
Fig. 1 application fields and typical cases of ultrasonic machining
“A sharp tool is necessary for doing a good job.” Ultrasonic machining technology is a sharp tool for precision machining of difficult materials. In most cutting fields, ultrasonic machining is referred to as “ultrasonic assisted precision machining,” which means using ultrasonic vibration to enhance traditional cutting technology and achieve a special material removal effect. However, in some cases, ultrasonic vibration becomes the main or even the only cutting power, and this type of ultrasonic machining can be referred to as direct ultrasonic machining.
For instance, the ultrasonic scalpel used in the medical field for bone cutting and the ultrasonic Dagger Knife used in aviation honeycomb part processing are examples of direct ultrasonic machining.
In the 1920s, scientists from the United States, Japan, Germany, and the Soviet Union began the basic research of vibration machining, with a focus on improving cutting conditions to break material chips. This early research was mainly in the field of ultrasonic turning and featured low-frequency vibration machining, which had a frequency quite different from the current ultrasonic frequency of above 15KHz.
In the early 2000s, due to the increasing number of difficult-to-process materials, universities and research institutes in China began research on ultrasonic machining technology. This research covers equipment design, control technology, ultrasonic machining systems, and ultrasonic technology.
Ultrasonic machining technology has gone through a budding stage from its inception to about 2000 and then a rapid development stage for more than 20 years. In the past five years, the rapid and large-scale application of difficult-to-process materials has accelerated the commercial development of ultrasonic machining technology.
Currently, ultrasonic machining equipment, ultrasonic vibration control, and technology are gradually maturing both domestically and internationally, which also deepens people’s understanding of the basic requirements, working mechanism, process characteristics, and application fields of ultrasonic machining technology.
Moreover, more and more universities and research institutes are conducting research on ultrasonic machining technology, and the application fields of ultrasonic machining are expanding.
Currently, the understanding of ultrasonic machining technology is still evolving, and there is no unified standard or specification both domestically and internationally.
The conventional ultrasonic machining system typically consists of an ultrasonic drive controller, ultrasonic transmitter, transducer, horn, clamping structure, and cutter, as shown in Figure 2.
This article will cover the characteristics of ultrasonic machining technology through the classification of the ultrasonic machining system, the typical structure of the ultrasonic machining system, ultrasonic drive control technology, and ultrasonic process technology.
Fig. 2 main components of ultrasonic machining system
Ultrasonic machining can be classified based on the form of ultrasonic vibration into one-dimensional, two-dimensional, and three-dimensional ultrasonic machining. The vibration forms include longitudinal (axial) ultrasonic vibration machining, torsional ultrasonic vibration machining, elliptical ultrasonic vibration machining, and composite ultrasonic vibration machining, with the latter involving a combination of different ultrasonic vibration forms.
According to the vibration starting materials, ultrasonic machining can be divided into electrostrictive ultrasonic machining and magnetostrictive ultrasonic machining. The former is based on the vibration starting principle of piezoelectric ceramics, driven by applying voltage, with a simple structure and mature technology but limited vibration power, making it more suitable for light load cutting conditions. The latter is based on the magnetic field driving principle of magnetostrictive or giant magnetostrictive materials, with a large vibration power capacity but a complex structure, making it more suitable for heavy-duty cutting conditions.
In terms of energy transmission mode, ultrasonic machining can be divided into wired energy transmission ultrasonic machining and wireless energy transmission ultrasonic machining. The former is often used in low-speed machining without rotating motion or using brushes, while the latter is generally used in rotary ultrasonic machining. The wireless energy transmission is realized through the loose coupling non-contact method and can be further divided into fully coupled ultrasonic machining and partially coupled ultrasonic machining. The latter is the most adaptable for automatic tool change of machine tools at present.
According to process types, ultrasonic machining can be divided into ultrasonic milling, ultrasonic turning, ultrasonic drilling, ultrasonic grinding, ultrasonic polishing, ultrasonic cutting, and ultrasonic hardening (strengthening). Each process has specific requirements for the size of ultrasonic energy and vibration form.
In terms of machining accuracy, ultrasonic machining can be divided into precision ultrasonic machining and ultra precision ultrasonic machining. The difference between the two lies in the amount of material removal per unit cutting, with removal accuracy requirements less than 1μm considered as ultra precision ultrasonic machining. Elliptical ultrasonic machining is typically used for micro nano cutting.
Finally, ultrasonic machining can be divided into traditional ultrasonic machining and high-speed ultrasonic machining. The latter refers to ultrasonic machining with a linear speed of up to 400m/min.
A typical ultrasonic machining machine tool is illustrated in Figure 3. It consists of an ultrasonic drive controller, a wireless energy transmission module, an ultrasonic tool holder, a tool, a numerical control system, and a machine tool body.
The ultrasonic drive controller comprises an ultrasonic generator, an ultrasonic power amplifier, an ultrasonic feedback detection, and an ultrasonic controller. The ultrasonic transmitter and the ultrasonic receiver make up the wireless energy transmission module.
The ultrasonic tool holder is the key functional component of the ultrasonic machining system, typically composed of the ultrasonic receiver, transducer, and horn. The ultrasonic machining system and the machine tool’s CNC system should have a certain level of communication control to ensure the smooth progression of the entire machining process.
Fig. 3 structure of typical ultrasonic machining machine
The driving control technology of the ultrasonic machining system is critical to realizing the benefits of ultrasonic machining technology, and the performance of the controller is the key to evaluating the performance of ultrasonic machining.
In the machining process, the ultrasonic drive controller must control numerous parameters, including the most important ultrasonic frequency and power, as well as the frequency resolution, response speed, and amplitude fluctuation of the ultrasonic machining system. These parameters are crucial in determining the effectiveness of the material processing.
The cutting process of tools into and out of materials is a typical strong time-varying load process, meaning that the cutting force changes significantly in a short period. In this process, the impedance characteristics of the ultrasonic machining system and the electrical characteristics of the control system will undergo significant changes, making it essential to maintain stability in the amplitude.
The frequency resolution and accuracy of the ultrasonic controller and the system’s response speed are critical parameters in this process. Figure 4 shows the change in system characteristics during a typical ultrasonic cutting process. As shown in the figure, when the tool begins to enter the machining state, the cutting force increases instantly. To ensure the stability of the ultrasonic amplitude during the cutting process, the system adjusts the internal control parameters (such as frequency and power) to maintain a stable vibration amplitude during the machining process. A similar process occurs when the tool cuts out the material.
Fig. 4 change of state characteristics of ultrasonic machining system
During ultrasonic machining, the changes in load and temperature result in significant changes in the system characteristics during the machining process. Fast frequency tracking is crucial for effective ultrasonic machining, and common methods include the maximum current method, phase locked loop method, and maximum power method.
Ultrasonic power adaptive control technology is also an important aspect of ultrasonic machining, aimed at resolving the issue of the tool being unable to complete effective cutting due to suppressed amplitude after loading. These tests require the ultrasonic machining system to have real-time feedback detection of the ultrasonic actuator’s state. Therefore, feedback detection of ultrasonic vibration is a crucial part of achieving stable ultrasonic machining.
Nowadays, advanced algorithms are being applied in this process, including PID, fuzzy algorithms, and artificial neural networks. The core of these algorithms is to identify the state during the machining process, ensuring the robustness of the machining process.
For materials with varying properties, the mechanism of material removal and the effect of ultrasonic machining can vary greatly.
Hard working materials can be classified into three categories: hard and brittle materials, composite materials, and hard-to-machine metal materials.
Hard and brittle materials, such as glass, ceramics, tungsten steel, and ceramic-based materials, are typically processed with diamond tools. During processing, the hardness of the material must be considered, and efforts must be made to reduce the cutting force and improve processing quality through ultrasonic processing.
Composite materials, such as carbon fiber reinforced composites, aramid fiber composites, and honeycomb composites with low stiffness, require the selection of appropriate ultrasonic vibration forms and amplitudes based on their material properties to reduce the cutting force and slow down tool wear.
Metal materials that are difficult to machine, including titanium alloys, high-temperature alloys, high-strength steels, and other metals with high toughness, require solving the problem of tool adhesion, reducing cutting temperature, and slowing down tool wear. The ultrasonic vibration requirements for these materials differ greatly from those for hard and brittle materials, and torsional vibration or longitudinal torsion is often used, with relatively high amplitude requirements.
For metal materials, the ideal machining scenario involves complete intermittent cutting of the material, efficient cooling and lubrication in the cutting area.
The following summarizes the main process characteristics of different materials during ultrasonic machining.
(1) Hard Brittle Materials:
Materials such as glass, ceramics (alumina, zirconia, silicon carbide, and silicon nitride), ceramic-based materials, glass ceramics, and other materials with high hardness and brittleness are considered hard brittle materials.
The main challenges in processing these materials include significant surface damage, rapid tool wear, and low processing efficiency.
Ultrasonic machining can help to improve the cutting state, leading to longer tool life, better surface quality, and increased machining efficiency.
Figure 5 shows a comparison of the average cutting force between ultrasonic machining and conventional machining of semiconductor silicon carbide (SIC).
Fig. 5 Comparison of average cutting force between ultrasonic machining and ordinary machining of silicon carbide
(2) Composite Materials:
Carbon fiber-reinforced and aramid fiber-reinforced composites are prone to surface damage, tearing, delamination, low processing efficiency, and rapid tool wear during processing.
However, ultrasonic machining can significantly enhance the cutting ability of the tool, reduce burrs, and extend the tool’s service life.
Figure 6 shows the result of ultrasonic machining on aviation honeycomb material.
a) Disc cutter machining
b) straight edge cutter machining
Fig. 6 ultrasonic machining of aviation honeycomb materials
(3) Difficult-to-Machine Metal Materials:
Metal materials with a certain level of toughness, such as titanium alloys, high-temperature alloys, and high-strength steels, are prone to issues such as tool adhesion and rapid tool wear due to high processing temperatures.
Ultrasonic machining can reduce cutting force, lower cutting temperature, improve chip shape, reduce tool adhesion, and extend tool life.
Figure 7 shows a comparison of tool wear between ultrasonic machining and conventional machining of titanium alloys.
a) Comparison of cutting tools under different removal amounts
b) Comparison and improvement ratio of tool wear under different removal amounts
Fig. 7 Comparison of tool wear between ultrasonic machining and ordinary machining of titanium alloy
Currently, ultrasonic machining technology is rapidly advancing.
The availability of various new materials provides a solid foundation for the application of ultrasonic machining.
As a result, ultrasonic machining has become a powerful tool for precision machining of these materials.
In the future, ultrasonic machining technology will continue to rapidly develop as the industry advances.
At the same time, the following research areas are likely to become the focus of future scientific research and engineering applications.
In the future, multi-dimensional ultrasonic machining technology will be increasingly utilized, and the development of corresponding control technology and process technology will be a key area of research.
Furthermore, the integration of ultrasonic, laser, plasma, and other processes into a composite technology is a crucial direction for the future development of ultrasonic machining.
The use of multiple energy fields in the processing of difficult-to-machine materials will bring unique benefits, making the processing easier.
The actual cutting process is distinct from static or quasi-static processes.
Variations in materials, tools, and cutting parameters can have a significant impact on the cutting force, cutting temperature, and system impedance characteristics during machining. The strong time-varying load can also cause instability in the ultrasonic machining system.
To achieve efficient and precise machining, it is crucial to ensure the stability of the ultrasonic machining system through fast, accurate, and stable control algorithms.
In the future, more advanced control algorithms will be incorporated into the ultrasonic machining process.
In traditional precision machining, it can be challenging to achieve both high machining quality and efficiency, especially when machining complex structural parts in the aerospace industry.
To ensure machining quality, the machining efficiency is often compromised.
However, when ultrasonic machining is applied to process difficult-to-machine materials in this field, such as titanium alloys, superalloys, and carbon fiber composite materials, its unique intermittent cutting mode allows for periodic opening of the cutting area during the cutting process, improving cooling and lubrication, reducing cutting force and temperature, and increasing cutting speed. As a result, high-quality and efficient precision machining can be achieved.
The process capability of ultrasonic machining is a clear demonstration of its advantages.
A thorough understanding of the process capability can help to fully utilize the capabilities of ultrasonic machining.
As technical research advances, more specialized tools, fixtures, and matching process parameters for ultrasonic machining will become available, allowing for greater utilization of its capabilities.
However, at present, there is no unified standard for ultrasonic machining technology.
The saying goes, “To know the straightness, you must follow the standard; to know the surroundings, you must follow the rules.
Accelerating the development of industrial and national standards will help to remove technical barriers and promote the widespread adoption and development of ultrasonic machining technology.
It’s important to note that ultrasonic machining technology is not a one-size-fits-all solution for difficult-to-machine materials.
Different types of ultrasonic machining have their own strengths and limitations, and it is crucial to be familiar with the technology’s characteristics and understand the process requirements for optimal application.
Ultrasonic machining technology is a cutting-edge manufacturing technology with tremendous growth potential, and it serves as a crucial technical support for manufacturing power.
As the use of difficult-to-machine materials increases, ultrasonic machining technology will be rapidly adopted and developed.
In the future, ultrasonic machining technology will provide solutions to precision machining problems for an even wider range of difficult-to-machine materials.