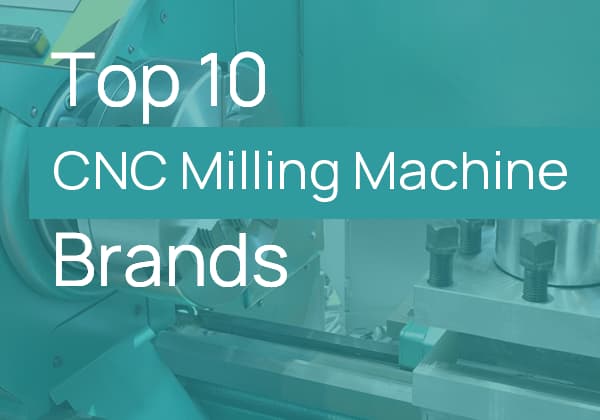
Have you ever wondered how tiny holes are drilled with such precision in metal? This article explores the fascinating world of drilling, revealing the secrets behind various drill bits and techniques. You’ll learn how professionals achieve perfect holes and the tools they use. Get ready to uncover the intricacies of drilling!
Various cutting processes of drilling, reaming, or counterboring are done using different types of drill bits.
Drilling is a cutting process that produces holes by using twist drills, flat drills, or center drills on solid materials to create through-holes or blind holes.
Reaming enlarges the diameter of a pre-existing hole in a workpiece using a reamer drill.
Counterboring is performed using a counterbore drill at one end of the pre-existing hole to produce counterbores, tapered holes, partial planes, or spherical shapes, which are used for installing fasteners.
There are two main methods of drilling:
1) the workpiece remains stationary while the drill bit rotates and advances axially, which is generally applied on drilling machines, boring machines, machining centers, or combination machine tools;
2) the workpiece rotates while the drill bit only advances axially, which is generally applied on lathes or deep hole drilling machines. Twist drills can produce hole diameters ranging from 0.05mm to 100mm, while flat drills can achieve up to 125mm. For holes larger than 100mm, a smaller pre-drilled hole (or reserved casting hole) is usually made first, and then the hole is bored to the required size.
During drilling, the drilling speed (v) is the circumferential speed of the drill bit’s outer diameter (m/min), and the feed rate (f) is the axial distance that the drill bit (or workpiece) moves per revolution while drilling into the hole (mm/r).
Figure 2 shows the drilling parameters of a twist drill. As a twist drill has two cutting edges, the feed rate for each tooth is calculated as af=f/2 (mm/tooth).
There are two cutting depths: when drilling holes, it is calculated as half of the drill bit diameter (d); when reaming, it is calculated as (d-d0)/2, where d0 is the pre-existing hole diameter.
The chip thickness cut by each tooth is a0=afsin(Κr), with units in millimeters, where Κr is half of the drill bit’s point angle.
When using high-speed steel twist drills to drill steel materials, the drilling speed is usually set between 16-40 m/min; using hard alloy drill bits can double the drilling speed.
During the drilling process, a twist drill has two primary cutting edges and one transverse edge, commonly referred to as “one point (drill center) and three blades”, which participate in cutting.
The twist drill works in a semi-enclosed state where the transverse edge is severely squeezed and chip removal is difficult. Therefore, the processing conditions are more complex and challenging than turning or other cutting methods, resulting in lower processing accuracy and rougher surfaces.
The precision of drilling steel materials is generally IT13-10, with surface roughness of Ra20-1.25μm, while the precision of reaming can reach IT10-9, with surface roughness of Ra10-0.63μm.
The quality and efficiency of the drilling process largely depend on the shape of the cutting edge of the drill bit.
In production, the shape and angle of the cutting edge of a twist drill are often changed by sharpening to reduce cutting resistance and improve drilling performance. China’s group drill is an example of a twist drill produced using this method.
When the ratio of depth (l) to diameter (d) of a drilled hole is greater than six, it is generally considered deep hole drilling. The drill bit used for deep hole drilling is slender and has poor rigidity. During drilling, the drill bit is prone to deviation and friction with the hole wall, making it difficult for cooling and chip removal.
Therefore, when the l/d ratio is greater than 20, a specially designed deep hole drill bit is required and a cutting fluid with a certain flow rate and pressure is used for cooling and chip flushing to achieve high-quality drilling results with high efficiency.
A drill bit is a cutting tool used for drilling holes in solid materials, either to create through holes or blind holes, and can also be used to enlarge existing holes.
Commonly used drill bits include twist drills, flat drills, center drills, deep hole drills, and counterbore drills. Although reamers and countersinks are not used to drill holes in solid materials, they are often classified as drill bits.
Figure 3. Various types of drill bits.
Twist drills are the most widely used hole processing tools. The diameter ranges from 0.25mm to 80mm. It is mainly composed of a working part and a shank part.
The working part has two helical grooves resembling a twisted ribbon, which is why it is called a twist drill. In order to reduce the friction between the guiding part and the hole wall during drilling, the diameter of the twist drill gradually decreases from the tip to the shank in a tapered shape.
The spiral angle of the twist drill mainly affects the size of the front angle of the cutting edge, the strength of the edge blade, and the chip removal performance, usually ranging from 25° to 32°.
The spiral groove can be processed by milling, grinding, hot rolling, or hot extrusion, and the cutting part of the drill bit is formed after being sharpened.
The top angle of the cutting part of a standard twist drill is 118°, the transverse edge inclination angle is 40° to 60°, and the back angle is 8° to 20°. Due to structural reasons, the front angle is gradually reduced from the outer edge to the middle, and there is a negative front angle (up to about -55°) at the transverse edge, which exerts a pressing effect during drilling.
In order to improve the cutting performance of the twist drill, the cutting part can be ground into various shapes (such as group drills) according to the properties of the material being processed. The shank of a twist drill has two forms: straight shank and taper shank. During processing, the former is clamped in the drill chuck, and the latter is inserted into the tapered hole of the machine tool spindle or tailstock.
Generally, twist drills are made of high-speed steel. Twist drills with cemented carbide blades or teeth are suitable for processing cast iron, hardened steel, non-metallic materials, etc., and solid carbide small twist drills are used for processing instrument parts and printed circuit boards, etc.
The cutting part of the flat drill is shovel-shaped, and its structure is simple with a low manufacturing cost. The cutting fluid can be easily introduced into the hole, but its cutting and chip removal performance is poor. Flat drills can be divided into two types: integral and assembled.
The integral type is mainly used for drilling micro-holes with a diameter of 0.03mm to 0.5mm. Assembled flat drills have replaceable blades and can be internally cooled. They are mainly used for drilling large holes with a diameter of 25mm to 500mm.
Deep hole drills usually refer to tools that have a hole depth-to-diameter ratio greater than 6. Commonly used deep hole drills include gun drills, BTA deep hole drills, jet drills, DF deep hole drills, etc. Counterbore drills are also commonly used for deep hole processing.
Reamers have 3-4 teeth and are more rigid than twist drills. They are used to enlarge existing holes and improve processing precision and smoothness.
Counterbore drills have multiple teeth and are used to shape the end of holes, such as counterbore holes for various types of countersunk screws or to flatten the outer end surface of holes.
Center drills are used to drill center holes in shaft-type workpieces. Essentially, they are composed of twist drills and counterbore drills with very small helix angles, and are also called compound center drills.
When mechanical process personnel choose a drill for a specific hole processing task, the depth of the processed hole needs to be considered first. The deeper the processed hole is, the more chips need to be discharged during the machining process.
If the chips generated during processing cannot be discharged timely and effectively, it may block the chip removal groove of the drill, thereby delaying the machining process and ultimately affecting the quality of the hole processing.
Therefore, effective chip removal is a key factor in successfully completing any material’s hole processing task.
When process personnel choose the most suitable type of drill bit for a specific hole processing task, they need to calculate the length-to-diameter ratio of the drill bit.
The length-to-diameter ratio is the ratio of the depth of the processed hole to the diameter of the drill bit. For example, if the diameter of the drill bit is 12.7mm and the depth of the hole to be machined is 38.1mm, then its length-to-diameter ratio is 3:1.
When the length-to-diameter ratio is about 4:1 or less, most standard twist drill bits can smoothly discharge the chips cut by the cutting edge of the drill bit.
However, when the length-to-diameter ratio exceeds the above range, specially designed deep hole drill bits are required to achieve effective machining.
Once the length-to-diameter ratio of the processed hole exceeds 4:1, it is difficult for standard twist drills to lift chips off the cutting area and discharge them outside the hole. Chips will quickly block the chip removal groove of the drill bit.
At this point, it’s necessary to stop drilling, retract the drill bit from the hole, clear the chips from the chip removal groove, and then resume drilling to continue cutting.
The above operation needs to be repeated many times to achieve the required depth of the hole. This drilling method is usually called “peck drilling”. Using “peck drilling” to machine deep holes will shorten the tool life, reduce machining efficiency, and affect the quality of the processed hole.
Every time the drill bit is retracted from the hole to clear the chips and reinserted into the hole, it may deviate from the centerline of the hole, causing the hole diameter to increase beyond the specified size tolerance range.
In order to solve the problem of deep hole machining, drill bit manufacturers have developed two new types of deep hole machining drill bits in recent years – ordinary parabolic drill bits and wide-blade parabolic drill bits.
The chip removal groove of a parabolic drill bit is parabolic in shape and is specifically used for continuous drilling of deep holes with a length-to-diameter ratio of up to 15:1 and material hardness of not more than 25-26 HRC (including low carbon steel, various aluminum alloys, copper alloys, etc.).
For example, a parabolic drill bit with a diameter of 12.7mm can successfully machine a hole depth of up to 190mm.
Due to its large chip removal space, an ordinary parabolic drill bit can quickly discharge the chips at the cutting edge while allowing more cutting fluid to enter the cutting area, thus significantly reducing the possibility of cutting friction and chip welding.
Additionally, it also reduces power consumption, torque load, and cutting impact during machining.
The helix angle of a parabolic drill bit is 36°-38°, which is greater than the helix angle of a standard twist drill (28°-30°). The helix angle can indicate the degree of “twist” of the drill bit, and the larger the helix angle, the faster the drill bit and chip removal speed.
Another characteristic of ordinary parabolic drill bits suitable for deep hole machining is that the drill core is thicker (the drill core of the drill bit refers to the center part of the drill bit that has not been ground after the chip removal groove is formed).
The drill core of a standard twist drill accounts for about 20% of the entire finished drill bit, while the drill core of a parabolic drill bit can account for about 40% of the entire drill bit.
In deep hole drilling, a thicker drill core can increase the rigidity of the drill bit and improve the stability of the drilling process. The drill tip of the parabolic drill bit has a slot, so a larger drill core diameter can be used. Additionally, it can prevent the drill bit from shifting during the initial stage of drilling.
Parabolic drill bits are made of high-speed steel and can be surface coated to enhance their cutting performance.
To meet the needs of deep hole drilling of difficult-to-machine materials (such as cold-worked hardened materials), some tool manufacturers have developed wide-blade parabolic drills.
Many features of this type of drill bit are similar to those of ordinary parabolic drills, such as a larger helix angle (36°-38°) for easy chip removal and a thicker drill core for better rigidity and stability during deep hole machining.
The difference between it and ordinary parabolic drill bits lies in the shape of the chip removal groove and the blade edge. The blade edge of the wide-blade parabolic drill bit smoothly transitions into the chip removal groove, making the cutting edge of the drill bit stronger and more rigid. At the same time, chips can be smoothly discharged through the chip removal groove.
In deep hole drilling, the high temperature caused by friction may cause slight softening or annealing of the cutting edge of the drill bit, which accelerates its wear. The ability of the cutting edge of the drill bit to maintain hardness during processing can be expressed as “red hardness”.
Wide-blade parabolic drills are usually made of high-speed steel and cobalt high-speed steel materials. Due to the higher red hardness of cobalt high-speed steel, the tool life is longer and the resistance to wear is stronger.
The following surface coatings are commonly used for ordinary parabolic drills and wide-blade parabolic drills:
① Titanium nitride (TiN) coating: This coating can significantly improve the service life of drill bits and the quality of processed holes. Compared with uncoated drill bits, TiN-coated drill bits are more suitable for high-speed drilling of various materials (especially various steel parts).
② Titanium carbonitride (TiCN) coating: At the appropriate cutting temperature, TiCN-coated drill bits have higher hardness, stronger toughness, and better wear resistance than TiN-coated drill bits. They are also suitable for high-speed drilling of various materials (especially steel parts).
However, they should be used with caution when processing non-ferrous metal materials because the TiCN coating has a high chemical affinity with non-ferrous metals and is prone to wear.
③ Titanium aluminum nitride (TiAlN) coating: This coating can improve the service life of drill bits, especially in high-temperature cutting environments. Similar to TiCN coating, TiAlN coating is not very suitable for processing non-ferrous metal materials.
In deep hole machining, in order to maximize the cutting performance of the drill bit, the drilling speed and feed rate must be optimized based on the specific length-to-diameter ratio.
When the length-to-diameter ratio of the drilling process is 4:1, the cutting speed should be reduced by 20%, and the feed rate should be reduced by 10%.
When the length-to-diameter ratio is 5:1, the cutting speed should be reduced by 30%, and the feed rate should be reduced by 20%. When the length-to-diameter ratio reaches 6:1-8:1, the cutting speed should be reduced by 40%. Additionally, when the length-to-diameter ratio is 5:1-8:1, the feed rate should be reduced by 20%.
Although the price of a parabolic drill bit is 2-3 times that of a standard twist drill bit, its excellent performance in deep hole (length-to-diameter ratio greater than 4:1) machining significantly reduces the cost of each hole drilled, making it the preferred tool for mechanical technicians to process deep holes.