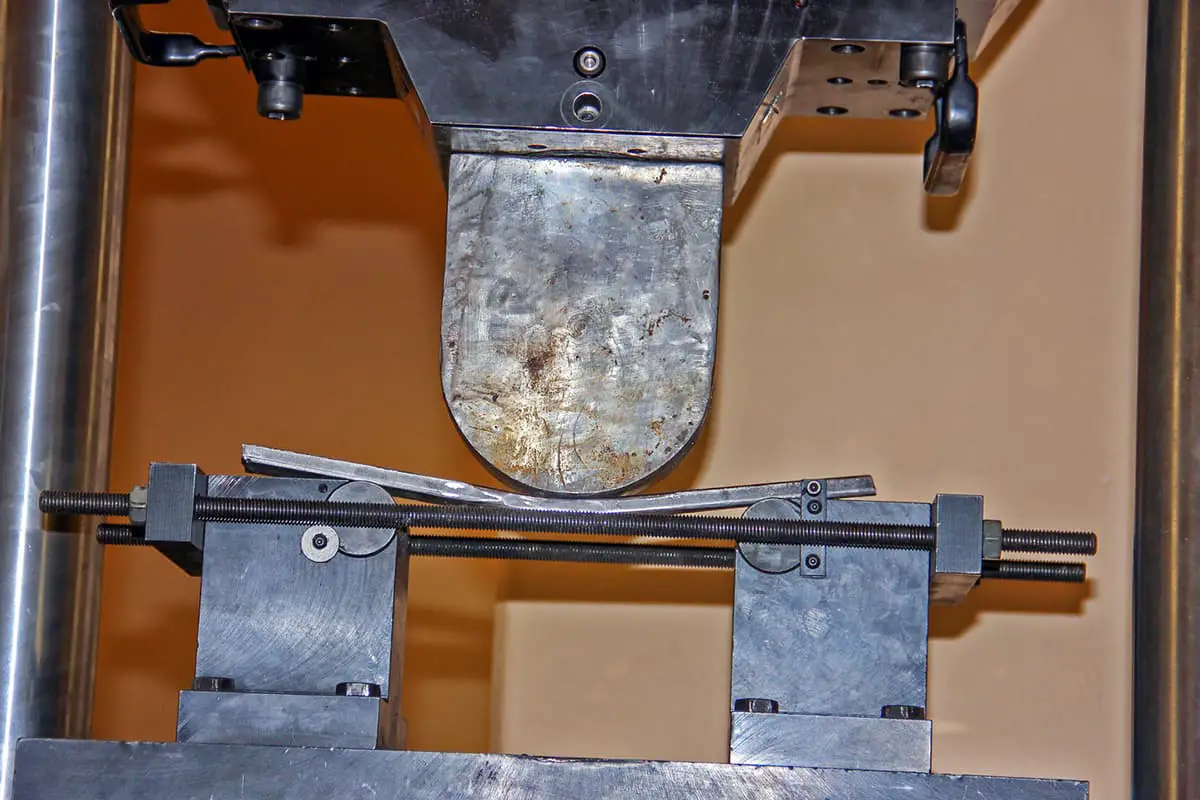
What makes one metal break under tension while another merely bends? This article explores the crucial differences between yield strength and tensile strength, explaining how each property affects material performance. Readers will learn about the factors influencing these strengths and their practical engineering implications, essential for selecting the right materials for various applications.
Three commonly used yield criteria in engineering are:
(1) Proportional Limit – The highest stress that maintains a linear relationship on the stress-strain curve, internationally represented as σp. Material is considered to start yielding when stress exceeds σp.
(2) Elastic Limit – After loading and unloading a test sample, the standard is no residual permanent deformation. The highest stress at which the material can fully recover elastically is commonly represented as σel internationally. Material is considered to start yielding when stress exceeds σel.
(3) Yield Strength – The standard is a specific residual deformation, such as 0.2% residual deformation stress taken as yield strength, symbolized as σ0.2 or σys.
The intrinsic factors affecting yield strength include:
Bonding, microstructure, structure, atomic properties. Comparing the yield strength of metal with ceramics and polymers demonstrates the fundamental impact of bonding.
From the perspective of microstructural influences, four strengthening mechanisms can affect the yield strength of metal materials:
(1) Solid solution strengthening;
(2) Strain hardening;
(3) Precipitation strengthening and dispersion strengthening;
(4) Grain boundary and subgrain strengthening.
Precipitation strengthening and grain refinement are the most common methods for improving yield strength in industrial alloys. Among these strengthening mechanisms, the first three decrease plasticity while improving material strength. Only refining grains and subgrains can increase both strength and plasticity.
The extrinsic factors affecting yield strength include:
Temperature, strain rate, stress state. As temperature decreases and strain rate increases, the material’s yield strength rises. Body-centered cubic metals are particularly sensitive to temperature and strain rate, leading to the phenomenon of steel’s low-temperature brittleness.
The effect of stress state is also significant. Although yield strength reflects a fundamental property of the material, different stress states will result in different yield strengths. Typically, when we refer to the yield strength of a material, we are referring to its yield strength under unidirectional tension.
Traditional strength design methods use the yield strength as a standard for plastic materials, defining the allowable stress [σ]=σys/n, where the safety factor n is typically 2 or greater. For brittle materials, tensile strength is used as the standard, defining allowable stress [σ]=σb/n, where the safety factor n is typically 6.
It’s important to note that following traditional strength design methods will inevitably lead to an overemphasis on high yield strength materials. However, as material yield strength increases, the material’s fracture resistance decreases, increasing the risk of brittle fracture.
Yield strength not only has direct application significance but also roughly measures certain mechanical behaviors and process performance of materials in engineering.
For example, an increase in material yield strength makes it more sensitive to stress corrosion and hydrogen embrittlement. If the yield strength of a material is low, it has better cold forming and welding properties. Therefore, yield strength is an indispensable key indicator of material properties.
After a material begins to yield, continued deformation will cause work hardening.
The work hardening index n reflects the strain hardening of a material after it begins to yield and continues to deform, determining the maximum stress when necking begins to occur. n also determines the maximum uniform strain that a material can produce, a crucial value in cold forming processes.
For working parts, it is also required that materials have certain work hardening capabilities.
Otherwise, under occasional overloads, excessive plastic deformation will occur, potentially resulting in local uneven deformation or fracture.
Therefore, the work hardening capability of a material is a reliable guarantee for the safe use of parts.
Strain hardening is an essential means of enhancing material strength. Stainless steel has a large work hardening index n=0.5, thus resulting in a high uniform deformation amount.
Although the yield strength of stainless steel is not high, it can be significantly improved through cold deformation. High carbon steel wire, after lead bath isothermal treatment and drawing, can reach above 2000MPa.
However, traditional strain strengthening methods can only increase strength while significantly reducing plasticity. In some new materials being developed, it is noted that changes in the microstructure and its distribution can improve both strength and plasticity during deformation.
Tensile strength represents the resistance to fracture when materials do not exhibit necking. When brittle materials are used in product design, their allowable stress is based on tensile strength. What does tensile strength mean for general plastic materials?
Although tensile strength only represents the maximum uniform plastic deformation resistance, it indicates the material’s limit load-bearing capacity under static tension. The external load corresponding to the tensile strength σb is the maximum load that the specimen can withstand.
Even though necking is continuously developing and the actual stress is increasing, the external load is dropping rapidly.
The work consumed per unit volume of material from deformation to fracture under static tension is called static toughness. Strictly speaking, it should be the area under the true stress-strain curve.
For simplicity in engineering, it is approximated as: For plastic materials, static toughness is a comprehensive indicator of strength and plasticity.
Pure high-strength materials like spring steel do not have high static toughness, and low carbon steel with good plasticity does not have high static toughness either.
Only quenched and high-temperature tempered medium carbon (alloy) structural steel possesses the highest static toughness.
Hardness is not an independent basic property of metals. It refers to the ability of a metal to resist deformation or fracture on its surface within a small volume.