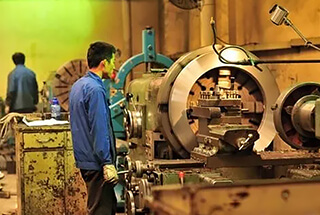
Are you tired of dealing with the mess and cost of cutting fluids in machining? Discover the benefits of dry machining—a process that eliminates the use of cutting fluids, reducing costs and environmental impact. This article explores how dry machining maintains high efficiency and quality in turning, milling, drilling, and boring operations. Learn about the latest tools and techniques that make this possible, and understand why industrialized nations are increasingly adopting this green manufacturing method. Dive in to see how dry machining can revolutionize your machining processes.
Currently, most machine parts processing, particularly on highly automated CNC machine tools, machining centers, and production lines, involves the use of cutting fluids. The primary functions of cutting fluids are chip removal, reducing cutting temperatures, and providing lubrication.
However, with increasing emphasis on environmental protection and sustainable development, the processing methods that heavily rely on cutting fluids are facing various restrictions.
The pollution from cutting fluids can harm the surrounding environment and the operators. Cleaning the residues that cutting fluids leave on parts and cutting surfaces not only causes “secondary pollution” but also increases production costs.
Research by the German VDMA and the Japan Society for Precision Engineering has shown that the cost of cutting fluids accounts for approximately 13% to 17% of the total manufacturing costs, while tool costs usually represent only 2% to 4%, as illustrated in the figure below.
Dry machining, in simple terms, is a machining process that doesn’t use any cutting fluids. Since the inception of metal cutting technology, both dry and wet cutting methods have been employed. Thus, the principle of dry machining is not new and has been applied in production for a considerable time (such as dry milling of cast iron).
Yet, the context has significantly changed because dry machining is no longer limited to the processing of cast iron materials and conventional natural methods. Instead, it strives to apply dry machining in the processing of all materials and methods through the establishment of new theories and techniques.
Dry machining is not merely about ceasing the use of cutting fluids. It’s about maintaining high efficiency, product quality, tool life, and reliability in the cutting process while minimizing or eliminating the use of cutting fluids. This requires the use of high-performance dry machining tools, machine tools, and auxiliary facilities to replace the role of cutting fluids in traditional cutting and achieve true dry machining.
Dry machining involves various aspects, such as tool materials, tool coatings, tool geometry, machining machines, cutting parameters, and processing methods. It represents an intersection and integration of manufacturing technology with material science, information technology, electronics, and management disciplines.
Dry machining aims to eliminate the adverse effects of cutting fluids in turning, milling, drilling, and boring processes, significantly reducing processing costs and protecting the ecological environment.
Currently, industrialized nations like those in Europe and Japan are paying great attention to the development and application of dry machining technology. Statistics show that about 10% to 15% of machining in the European industrial sector has adopted dry machining processes.
In the 21st century, the manufacturing industry’s demand for green and environmentally friendly processes is ever-increasing. Dry machining technology, as a green manufacturing process, is significant for resource conservation, environmental protection, and cost reduction.
With the advancement of machine tool technology, cutting tool technology, and related process research, dry machining is set to become a primary means of metal cutting and gain widespread application.
At present, the scope of dry machining processing is still relatively limited, but its in-depth research and extensive application have become a hot topic in the field of machining. Israeli experts believe that dry machining “remains a complex field to this day. It’s not just a matter of turning off the coolant and ordering a new tool.”
In recent years, alongside the development of high-speed cutting technology, the mechanical manufacturing industry in industrialized countries has been exploring new dry machining processes using existing tool materials.
Meaningful and economically viable dry machining should be based on a careful analysis of specific boundary conditions and a thorough understanding of the complex factors influencing dry machining. This analysis provides the necessary data and materials for the design of dry machining process systems.
Dry machining research and applications have received widespread attention both domestically and internationally, with years of research propelling the implementation of dry machining technology.
However, experts and scholars have varying descriptions of the terminology and definitions related to dry machining. The proposed national standard provides regulations: The standard applies to mechanical product machining processes that involve complete dry machining and assisted dry machining (including air cooling, liquid nitrogen cooling, laser-assisted, etc.).
Dry Machining (Dry cutting): A machining process that does not use any cutting fluid during the cutting process.
Complete Dry Machining: A machining process that does not use any cutting fluid or auxiliary cooling medium during the cutting process.
Sub-Dry Machining (Sub-dry cutting): A cutting technology that involves injecting an adequate amount of lubricant into a stream of air at certain pressures and temperatures, creating a mist mixture, which then forms a minimal quantity of lubricating medium and is sprayed into the cutting zone for targeted micro-lubrication and cooling of the heat-affected area.
Sub-dry machining usually includes: minimal quantity lubrication cutting, low-temperature minimal quantity lubrication cutting (cold air cutting), protective gas minimal quantity lubrication cutting, internal coolant jetting, and mixed spraying technologies.
The aforementioned terms and definitions provide a basic distinction and standardization between dry cutting and sub-dry cutting. As research deepens and technology advances, the standards also undergo a continuous process of improvement and refinement. For instance, in the definition of sub-dry cutting (sub-dry machining), “injecting an adequate amount of lubricant” should be revised to “injecting an adequate amount of cooling and lubricating medium” to form a mist mixture with air at certain pressures and temperatures, creating a micro-cooling lubricating medium that is sprayed into the cutting area for targeted micro-cooling and lubrication of the heat-affected zone.
Cooling Effect
The cooling effect removes heat generated during cutting, reduces tool wear, and prevents workpiece surface oxidation.
Lubricating Effect
It reduces friction, lowers cutting forces, and ensures smooth cutting operations.
Chip Removal
The process swiftly removes chips from the workpiece surface, preventing them from scratching the surface.
However, from an environmental protection standpoint, the negative impacts of cutting fluids are increasingly evident, as seen in the following aspects:
Additionally, extensive research into the cutting process has revealed that the traditional roles of cutting fluids in cooling, lubrication, and chip removal are not fully and effectively utilized in many machining processes, especially in high-speed cutting.
As a result, efforts are being made to reduce or eliminate the use of cutting fluids, adapting to clean production processes and reducing production costs.
Dry machining technology emerged under these circumstances as an advanced machining method. Adopting dry machining technology not only reduces environmental pollution from cutting fluids and improves working conditions for operators but also eliminates related expenses for cutting fluids and lowers the cost of chip recycling and disposal.
Dry machining technology imposes higher demands on machine tool and cutting tool technology. In recent years, industrially advanced countries have placed great emphasis on dry machining research. Dry cutting machining, as a new method, represents one of the future trends in metal cutting technology.
dry machining, which eliminates the use of cutting fluids, completely eradicates a series of negative effects associated with their use during machining.
Compared to wet cutting, dry machining offers the following advantages:
However, compared to wet cutting under the same conditions, dry machining has some disadvantages:
The material of the workpiece largely determines the feasibility of implementing dry machining. Enhancing the machinability of materials, and reducing the heat generated by deformation and friction during the cutting process, are technical measures for the development of dry machining.
For instance, easily machinable steels and cast irons have been developed. Workpiece materials differ significantly in thermal properties; dry machining requires a large heat capacity and low thermal conductivity. Therefore, large-mass parts are more suitable for dry machining than small-mass parts.
High cutting forces and temperatures are the main characteristics of dry machining. To reduce material adhesion and diffusion between the tool and the workpiece at high temperatures and to ensure a normal tool life, special attention should be paid to the proper match between tool materials and workpieces.
Once the tool is determined, suitable cutting parameters and other variables should be selected based on the specific machining process. In dry machining operations, high cutting speeds are recommended since they facilitate quicker chip removal and heat dissipation, which are highly beneficial for extending tool life.
Current dry machining technologies include low-temperature air cooling, high-speed dry machining, low-temperature dry machining, electrostatic cooling, minimum quantity lubrication (MQL), and near-dry machining techniques.
1. Excellent thermal hardness and wear resistance.
Dry machining typically generates much higher cutting temperatures than wet machining. Only cutting tool materials with high thermal hardness can effectively withstand the high temperatures of the cutting process and maintain good wear resistance. The hardness of the tool material should be at least four times that of the workpiece material.
2. Low friction coefficient.
Reducing the friction coefficient between the tool and the chips, as well as between the tool and the workpiece surface, can partially substitute for the lubricating effect of cutting fluids and suppress the rise in cutting temperature.
3. High toughness at elevated temperatures.
Cutting forces during dry machining are greater than those in wet machining, and the cutting conditions for dry machining are more challenging; therefore, tools require high toughness at elevated temperatures.
4. High thermochemical stability.
Under the high temperatures of dry machining, cutting tools must maintain high chemical stability to minimize the catalytic effects of heat on chemical reactions, thereby extending tool life.
5. Reasonably structured tool geometry and angles.
Properly structured tool geometry and angles can not only reduce cutting forces, prevent the formation of built-up edge, and lower cutting temperatures but also control the chip flow and breakage. The shape of the tool ensures smooth chip evacuation and facilitates heat dissipation.