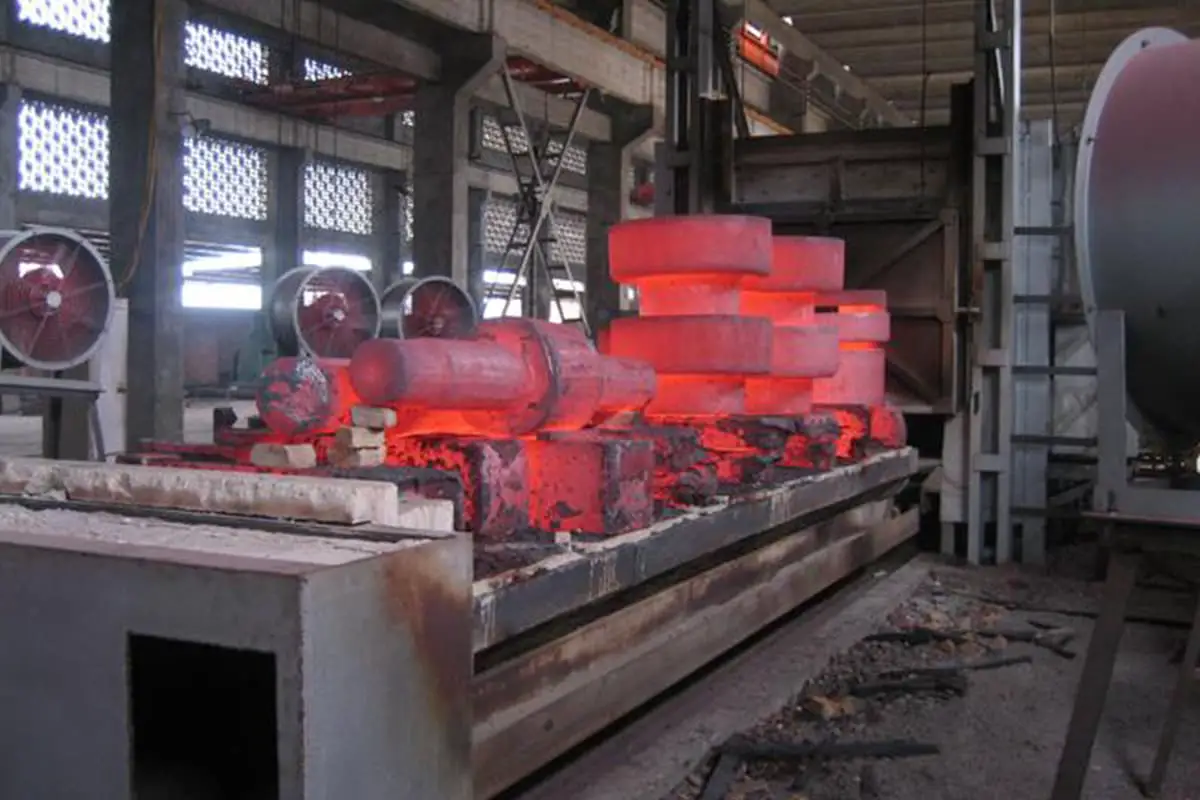
Have you ever wondered how metal parts achieve their desired properties? Annealing, a critical heat treatment process, holds the key. In this captivating article, we’ll dive into the world of annealing, exploring its various techniques and applications. Join us as we unravel the secrets behind this fascinating process that transforms the very structure of metals, unlocking their true potential.
Annealing is a metal heat treatment process that enhances the properties of the material by slowly heating the metal to a certain temperature, maintaining it for an adequate duration, and then cooling it at an appropriate rate. Depending on the objective and material characteristics, annealing techniques can be categorized into various types.
The purpose of annealing is to transform the pearlite in the steel after heating it to the austenitizing temperature.
After the annealing process, the structure of the material is close to being in a state of equilibrium.
Common types of annealing include:
Annealing Type | Description | Applications |
---|---|---|
Full Annealing | Mainly used for hypoeutectoid steels, such as medium carbon steel and low to medium carbon alloy structural steel forgings, castings, and hot-rolled sections. The purpose of full annealing is to refine the structure and reduce hardness. | – Medium carbon steel – Low to medium carbon alloy structural steel forgings – Castings – Hot-rolled sections |
Incomplete Annealing | Suitable for medium to high carbon steel and low alloy steel forgings and rolled parts. The degree of structure refinement is lower than that of full annealing. | – Medium to high carbon steel forgings and rolled parts – Low alloy steel forgings and rolled parts |
Isothermal Annealing | Suitable for situations where it is necessary to hold at a specific temperature for a period of time to achieve the desired effect. | – Specific applications requiring holding at a certain temperature |
Spheroidizing Annealing | Mainly used to improve the machinability and extend the service life of steel, suitable for bearing steel, etc. | – Bearing steel – Improving machinability and service life |
Stress Relief Annealing | Used to eliminate internal stresses after cold deformation while retaining the effect of cold work hardening. | – Eliminating internal stresses after cold deformation – Retaining cold work hardening effect |
Diffusion Annealing | Used to homogenize the chemical composition of alloy castings and improve their performance. | – Homogenizing chemical composition of alloy castings – Improving performance |
Laser Annealing, Traditional Oven Annealing, etc. | These are more modern or specific application annealing methods used to reduce hardness, improve machinability, eliminate residual stresses, stabilize dimensions, reduce deformation and cracking tendencies, refine grains, adjust structure, and eliminate structural defects. | – Reducing hardness – Improving machinability – Eliminating residual stresses – Stabilizing dimensions – Reducing deformation and cracking tendencies – Refining grains – Adjusting structure – Eliminating structural defects |
Induction Annealing and Contact Electric Brush Transmission of Large Current Annealing | These are methods that use electromagnetic induction principles or contact electric brush transmission of large currents to achieve annealing purposes. | – Specific applications requiring induction or large current annealing |
Thermal Annealing, Optical Annealing, Electron Beam Annealing, Laser Annealing, etc. | These are commonly used annealing processes for semiconductor materials, among which thermal annealing is the most commonly used. | – Semiconductor materials |
Process:
Heat the steel above Ac3 by 20 to 30 degrees Celsius, hold the temperature for a specified period of time, and then slowly cool it down (along with the furnace) to achieve a state close to equilibrium in the heat treatment process (fully austenitizing).
Complete annealing is mainly used for subeutectic steels (carbon content of 0.3 to 0.6%) such as medium carbon steel, low to medium carbon alloy steel castings, forgings, and hot-rolled profiles, and sometimes for their welds.
Low-carbon steel has low hardness and is not suitable for machining.
When hypereutectoid steel is heated above Accm to the austenitic state and annealed through slow cooling, Fe3CⅡ precipitates in a mesh pattern along the grain boundaries, significantly reducing the strength, hardness, plasticity, and toughness of the steel, which poses a potential risk to the final heat treatment.
Objective:
To achieve fine grain size, uniform structure, eliminate internal stress, reduce hardness, and improve machinability of steel.
The structure after complete annealing of hypoeutectic steel is F + P.
In order to increase efficiency in actual production, the parts are removed from the furnace for air cooling when the annealing temperature drops to around 500 degrees Celsius.
Complete annealing can take a long time, especially when dealing with highly stable austenitic steel.
If the austenitized steel is cooled to a temperature slightly lower than Ar1, resulting in a transformation from austenite to pearlite, followed by cooling to room temperature, it can greatly reduce the annealing time.
This method of annealing is called Isothermal Annealing.
Process:
Heat the steel to a temperature higher than Ac3 (or Ac1). After a specified period of heat treatment, it can be cooled to a specific temperature within the pearlite range, causing the austenitic structure to transform into pearlite, followed by cooling to room temperature.
Objective:
Similar to complete annealing, with easier control of the transformation process.
Suitable for steels with a more stable austenitic structure: high carbon steels (carbon content greater than 0.6%), alloy tool steels, high alloy steels (with more than 10% alloy elements).
Isothermal annealing can also help achieve uniform organization and performance.
However, it is not suitable for large section steel parts or large batch furnace materials because it is difficult to maintain the isothermal temperature throughout the internal or batch of workpieces.
The process of spherification annealing involves heating steel to a temperature between Ac1 and Ac3 (for hypoeutectic steel) or between Ac1 and Accm (for hypereutectic steel).
After holding the steel at the appropriate temperature for a set period of time, it is then slowly cooled to complete the heat treatment process.
This method of annealing is mainly used for hypereutectic steel to achieve a spherical pearlite structure, in order to reduce internal stress, lower hardness, and improve machinability. It is considered a type of incomplete annealing.
A heat treatment process for spheroidizing carbides in steel to obtain granular pearlite.
Process:
The steel is heated to a temperature that is 20-30℃ higher than Ac1, with a holding time of 2-4 hours. The cooling is usually done by a furnace method or isothermal at a temperature slightly below Ar1 for a long time.
This process is mainly used for eutectoid and hypereutectoid steels such as carbon tool steel, alloy tool steel, and bearing steel.
After rolling or forging, hypereutectoid steel forms lamellar pearlite and reticulated cementite which are hard and brittle, making them difficult to cut and prone to deformations and cracks during the quenching process.
Spheroidizing annealing forms globular pearlite in which the carbides appear as spherical particles dispersed in the ferrite matrix. This structure is low in hardness and easier to machine.
Additionally, the austenite grains are less likely to coarsen during heating and have less tendency to deform and crack during cooling.
It is important to normalize eutectic steel before spheroidizing annealing if it contains reticulated cementite to ensure that the spheroidizing process is successful.
Objective:
The goal of spheroidizing annealing is to reduce hardness, improve the uniformity of the structure, and improve machinability in preparation for quenching.
There are three main methods of spheroidizing annealing:
A) One-step spheroidizing annealing process:
The steel is heated to more than 20~30℃ above Ac1 and held for the appropriate time, then cooled slowly in the furnace. This process requires the original tissue to be finely laminated pearlite without any carburized networks.
B) Isothermal spheroidizing annealing process:
The steel is heated and insulated, then cooled to a temperature slightly below Ar1 and held isothermally (usually 10~30℃ below Ar1) before being cooled slowly in the furnace to about 500℃, then taken out for air cooling. This method has the advantages of short duration, uniform spheroidization, and easy quality control.
C) Reciprocating spheroidizing annealing process.
Process:
The ingots, castings, or forging billets are heated to a temperature slightly lower than the solid phase line for a prolonged period of time, then slowly cooled to eliminate unevenness in chemical composition.
Objective:
To eliminate dendritic segregation and regional segregation that occur during the solidification process, resulting in homogenization of composition and structure.
Diffusion annealing is conducted at very high temperatures, typically 100-200℃ above Ac3 or Accm, with the exact temperature depending on the severity of segregation and the type of steel. The holding time is typically 10-15 hours.
After diffusion annealing, the material must undergo complete annealing and normalizing to refine its structure. This process is applied to high-quality alloy steel and to alloy steel castings and ingots with serious segregation issues.
Process:
Heat the steel to a temperature below Ac1 (usually 500 to 650°C), hold it at that temperature, and then cool it in the furnace.
The stress-annealing temperature is lower than A1, so it does not cause any changes to the steel’s microstructure.
Objective:
To eliminate residual internal stress.
Recrystallization Annealing, also known as Intermediate Annealing, is a heat treatment process applied to metals that have undergone cold plastic deformation.
The objective of this process is to change the deformation grain into uniform and equal axial grains, which eliminates the process hardening and residual stress.
For recrystallization to occur, the metal must first undergo a certain amount of cold plastic deformation, and then it must be heated above a certain temperature known as the lowest recrystallization temperature.
The lowest recrystallization temperature for general metal materials is given below.
Trecrystallization = 0.4Tmolten
The recrystallization annealing temperature should be heated to a temperature that is 100 to 200℃ higher than the minimum recrystallization temperature (for steel, the minimum recrystallization temperature is approximately 450℃).
The annealing should be followed by proper heat preservation and a slow cooling process.
The following are the principles for selecting the annealing method:
(1) To decrease the hardness of steel, increase its plasticity, and make machining and cold deformation processing easier;
(2) To uniformly distribute the chemical composition and structure of the steel, refine the grain size, and improve its performance or prepare it for quenching;
(3) To eliminate internal stress and reverse the hardening effect caused by processing, thus avoiding deformation and cracking.
Both annealing and normalizing are mainly used as a preparatory step for heat treatment.
For parts with low stress and low performance requirements, annealing and normalizing can also serve as the final heat treatment.
When discussing annealing, it is essential to explore the materials that can be annealed, both metals and non-metals. This section will focus on the various materials that are commonly annealed.
Annealing plays a significant role in the processing of various metals and their alloys. Some of the widely used annealed metals include:
One commonly used method for annealing these materials is the use of car-bottom furnaces, which provide uniform heating and slow cooling essential for the annealing process.
Annealing is also appropriate for various non-metal materials, such as:
In conclusion, annealing is a vital process for a wide range of materials, including both metals and non-metals. By understanding the importance of annealing in different materials, we can better appreciate the role it plays in various industries.
According to the temperature used during heating, the commonly used annealing methods are categorized into:
Phase Change Recrystallization Annealing above the Critical Temperature (Ac1 or Ac3):
Annealing below the critical temperature (Ac1 or Ac3):
Complete annealing and incomplete annealing are two different heat treatment processes, differing in heating temperatures, structural transformations, grain refinement effects, and application scenarios.
Firstly, in terms of heating temperature, complete annealing typically heats the material above the critical temperature (Ac1 or Ac3) to promote phase change and recrystallization, while incomplete annealing involves heating in the two-phase region, preventing complete recrystallization. This implies that complete annealing can refine the grains of the material to a certain extent, but due to temperature constraints, incomplete annealing’s grain refinement effect is not as good as that of complete annealing.
Secondly, in terms of structural transformation, complete annealing can achieve a near-equilibrium structure, mainly used for medium carbon steel, etc., with the aim of refining grains, homogenizing structures, eliminating internal stress, reducing hardness, and so on. In contrast, incomplete annealing is primarily used for hypo-eutectoid steel to obtain a spherical pearlite structure, achieving a near-equilibrium structure through slow cooling.
Regarding grain refinement effects, due to the lower heating temperature of incomplete annealing, the form, size, and distribution of ferrite cannot change, and the grain refinement effect is not as good as complete annealing.
Lastly, in terms of application scenarios, complete annealing is suitable for situations that require grain refinement, structure homogenization, internal stress elimination, and hardness reduction, such as medium carbon steel. Incomplete annealing, on the other hand, is mainly used for hypo-eutectoid steel, especially when the grains have not coarsened, spherical pearlite structures can be obtained through incomplete annealing.
Isothermal annealing is a heat treatment process that involves heating the material above its critical temperature and maintaining it for a certain period, then cooling or holding it at another temperature. This process aims to refine the microstructure, reduce hardness, and improve material properties. The effects and limitations of this process vary across different materials.
For medium carbon alloy steel and low alloy steel, the purpose of isothermal annealing is to refine the structure and reduce hardness. The heating temperature for hypo-eutectoid steel is Ac3+(30~50)℃, and for hyper-eutectoid steel, it is Ac3+(20~40)℃. This indicates that isothermal annealing is suitable for these types of steel, effectively improving their mechanical properties.
However, isothermal annealing is not suitable for all situations. Sometimes, the availability of appropriate annealing equipment or the quality requirements of the annealed steel parts make continuous slow cooling the only feasible option. This means that in some cases, isothermal annealing may not meet specific heat treatment requirements.
Additionally, research on amorphous Cu56 Zr44 alloy indicates that isothermal annealing can be used for the crystallization process, altering the material’s microstructure. This suggests that isothermal annealing is also applicable to certain special materials, such as amorphous alloys. By proper temperature control and holding time, the expected crystallization effect can be achieved.
Stress relief annealing is a technique that eliminates residual internal stresses in workpieces through a process of heating, insulation, and slow cooling. This method is primarily used to alleviate internal stresses generated during welding, casting, and machining processes.
Specifically, the stress relief annealing process involves heating the workpiece to a lower temperature (for example, gray cast iron to 500-550°C, steel to 500-650°C), maintaining this for a certain period, and then slowly cooling to prevent the development of new residual stresses. While this treatment cannot completely eliminate the residual stresses within the workpiece, it can significantly reduce their impact.
Compared to traditional annealing methods, stress relief annealing has several advantages.
Firstly, it targets residual stresses specifically generated by certain manufacturing processes (like welding, casting, and machining) rather than broadly applying to all types of metal materials as traditional annealing does.
Secondly, stress relief annealing is typically carried out at lower temperatures, meaning it has less impact on the material, especially those sensitive to high temperatures.
Additionally, since the primary goal of stress relief annealing is to eliminate residual stress rather than merely reducing hardness or improving ductility, it can effectively reduce dimensional changes and tendencies towards cracking during the manufacturing process without significantly altering other physical properties of the material.
The comparative studies between laser annealing and traditional baking annealing in reducing hardness and improving machinability are mainly reflected in the following aspects:
Heating speed and control precision: The laser annealing technology has the characteristics of rapid heating and sensitive control, which enables it to reach the desired annealing temperature in a short time and precisely control the temperature changes during the annealing process. In contrast, traditional baking annealing requires the entire workpiece to be placed in a vacuum furnace and kept at a certain temperature range for a certain time, and the temperature control of this process is not as precise as laser annealing.
Heat conduction depth and energy output: Laser annealing technology can achieve localized and depth-controllable annealing treatment, which means that it can precisely heat treat specific areas as needed without affecting other areas. This localized heat treatment capability is very useful for improving the local performance of materials. Traditional baking annealing is difficult to achieve this localized heat treatment.
Grain refinement and microstructure adjustment: Laser annealing can cause atoms to rearrange through high temperature and thermal stress, making the crystal structure more orderly, which helps to increase the grain size and adjust the microstructure. This is beneficial for improving the machinability of materials and reducing hardness. Although traditional baking annealing can also refine grains and adjust the microstructure through the heating and cooling process, its process is relatively simple and direct, and it may not be able to precisely control the refinement of grains and the adjustment of the microstructure like laser annealing.
For example, in the preparation of Bi2Te3 stoichiometric thin films, the laser annealing method shows a higher Seebeck coefficient than the traditional thermal annealing method, proving its superiority in preparing high-quality thin films. This indicates that laser annealing technology may provide better performance in specific application fields (such as the preparation of high-performance thin films).