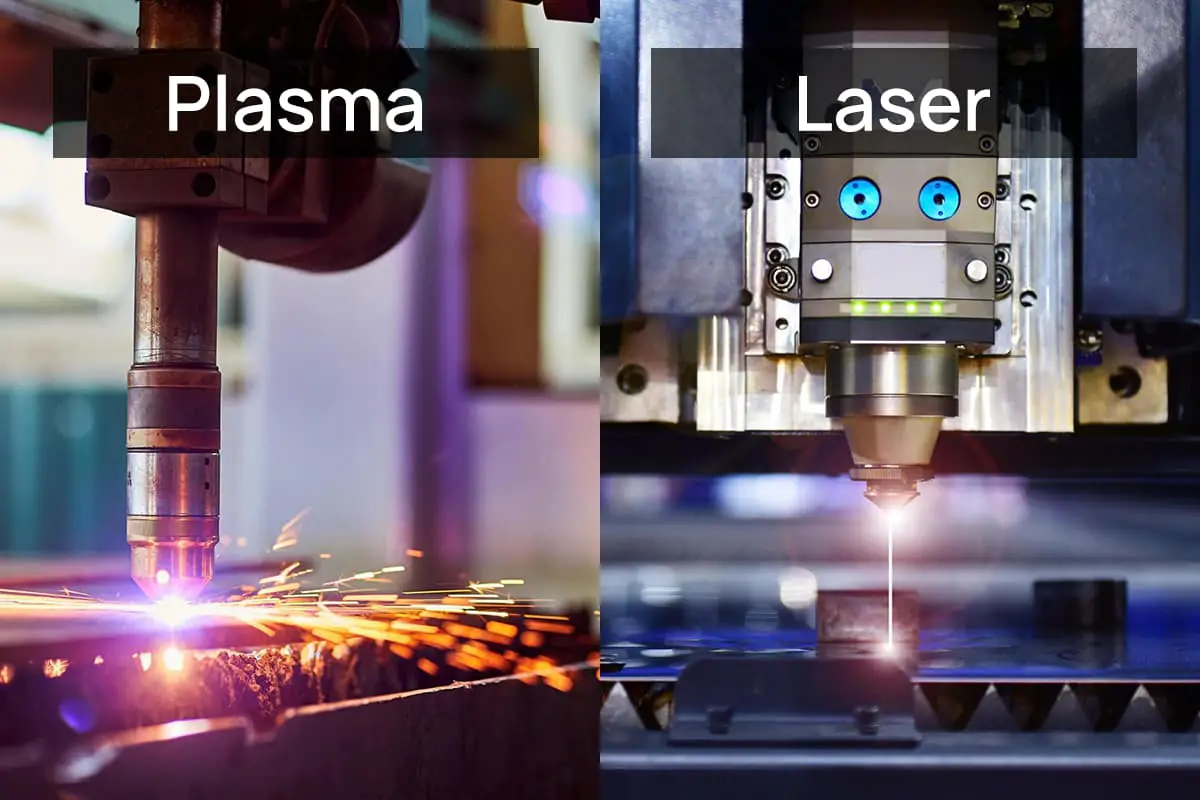
Why does laser cutting quality vary with the same equipment and parameters? This article dives into how the composition of carbon steel plates affects their cutting outcomes. By exploring the influence of elements like carbon, manganese, and chromium on cutting speed, surface roughness, and oxide formation, it highlights the intricate relationship between material properties and laser cutting performance. Readers will gain insights into optimizing cutting parameters for better results.
Laser cutting technology has gradually replaced traditional metal processing methods due to its low processing cost, fast production efficiency, precise cutting accuracy, and excellent processing quality. This technology is widely used in the processing of carbon steel, stainless steel, copper, aluminum, and other non-ferrous metals and is considered irreplaceable in these fields.
However, with the widespread use of laser cutting technology, some issues have arisen. One of the more significant problems is that when cutting carbon steel plates with the same thickness and different compositions using the same laser cutting machine and cutting process parameters, there are noticeable differences in the surface quality of the cuts.
To understand the impact of plate composition on cutting quality, tests were conducted on carbon steel plates with different thicknesses and compositions, using fiber lasers with power ranges of 6-30KW, and cutting with both oxygen and air.
Related reading: The Application of Air as Auxiliary Gas in Laser Cutting
The experiment was conducted using a 30KW fiber laser, which is a multimode continuous output laser with a beam wavelength of 1080nm and a core diameter of 150 μm. The laser head used in the experiment was the Genius 30 (30KW) laser head.
The quasi-diameter focal length of the laser head was 100mm, and the focusing mirror had a focal length of 200mm, which allowed for automatic focusing. To ensure stable operation of the 30KW fiber laser and maintain its optimal performance, a water cooler with a cooling capacity of 70.0KW was used as auxiliary equipment.
Fig. 1 – Experimental laser, laser head
To ensure the accuracy, efficiency, and clarity of the experimental data, the test materials used in this experiment were carbon steel plates of different thicknesses, including Q235, Q345, and Q460 carbon steel. For more details, please refer to the experimental plate data sheet.
The auxiliary gas used was 99.9% oxygen, with an air supply pressure of 5bar. To guarantee a sufficient number of nozzles, the nozzles listed in the experimental nozzle data sheet were prepared for the experiment.
Table 1 Data Sheet of Experimental Plate
Material type | Q235 | Q345 | Q460 | Q690 | NM400 | 45# | T10 |
Size/mm (L/W: 500/500) | 12 | 12 | 12 | 12 | 12 | 12 | 20 |
16 | 20 | 20 | 20 | 16 | 16 | 30 | |
20 | 30 | 30 | 30 | 20 | 20 | 40 | |
30 | / | / | / | 30 | 30 | / |
Table 2 Data Sheet of Experimental Nozzle
Nozzle type | Double jet | Single jet | ||||
Nozzle model | B-1 | B-2 | B-3 | D-4 | D-7 | D-9 |
Quantity | 5 | 5 | 5 | 5 | 5 | 5 |
Under the condition that the equipment’s spot quality (the optical fiber and laser head were clean and undamaged, verified through a photo paper test), air pressure (oxygen was maintained at a stable 5 bar, and air was maintained at a stable 11 bar), and internal lenses of the laser head (clean, free from dirt and burn marks) were normal, the internal modules were controlled through the laser’s internal control software, and the maximum output power was adjusted to 12KW, 20KW, and 30KW respectively.
Cutting experiments were performed on different types and thicknesses of plates described in Table 1, under the three power states mentioned above. The perimeter of the cut sample was 205.6mm, as shown in Fig. 2.
The cut samples were analyzed and compared by examining the porosity density, roughness, and corresponding process parameters on the surface of the cut samples.
Fig. 2 – Schematic Diagram of Cutting Sample
During the experiment, the five factors affecting the cutting speed (laser power, cutting gas pressure, focus, and nozzle aperture) were taken into consideration to ensure that the samples after cutting would automatically detach, with no slag, burning, droplets, and would have a high surface finish. The process parameters were adjusted to achieve the best cutting effect for different materials and thicknesses.
Related reading: Laser Cutting Thickness & Speed Chart
See Table 3 for specific parameters.
Table 3 Table of Experimental Parameters
Plate | Thickness/mm | Power | Speed m/min | Air pressure/bar | Power/KW | Focus/mm |
Q345B | 12 | 12KW | 1.7 | 0.8 | 9600 | 9.8 |
20 | 1.4 | 0.6 | 12000 | 11.6 | ||
30 | 0.9 | 0.7 | 12000 | 12.5 | ||
45# | 12 | 1.9 | 1.2 | 10000 | 9.8 | |
20 | 1.6 | 1.5 | 12000 | 9.5 | ||
30 | 1 | 0.6 | 12000 | 12.3 | ||
NM400 | 12 | 1.6 | 1 | 9000 | 9.6 | |
20 | 1.5 | 0.45 | 12000 | 12 | ||
30 | 1 | 1 | 12000 | 12 | ||
Q345B | 12 | 20KW | 1.7 | 0.8 | 9600 | 9.8 |
20 | 1.6 | 1.3 | 16000 | 12 | ||
30 | 1.2 | 1 | 17000 | 12.5 | ||
45# | 12 | 1.9 | 1.2 | 10000 | 9.8 | |
20 | 1.6 | 1.3 | 14000 | 12 | ||
30 | 1.2 | 1.5 | 16000 | 11.5 | ||
T10 | 20 | 1 | 1.4 | 15000 | 11 | |
30 | 0.8 | 1.6 | 18000 | 11.5 | ||
40 | 0.7 | 1.7 | 18000 | 11 | ||
NM400 | 12 | 1.6 | 1 | 9000 | 9.6 | |
16 | 1.8 | 0.55 | 14000 | 12 | ||
20 | 1.5 | 0.6 | 14000 | 12.5 | ||
30 | 1.1 | 0.85 | 17000 | 12.5 | ||
Q345B | 35 | 30KW | 1.3 | 1.8 | 30000 | 12 |
40 | 0.85 | 1.2 | 24000 | 12.5 | ||
45# | 30 | 1 | 3 | 26000 | 13 | |
40 | 0.8 | 1.7 | 30000 | 12.5 | ||
T10 | 35 | 0.8 | 1.6 | 18000 | 11.5 | |
40 | 0.7 | 1.7 | 18000 | 11 | ||
NM400 | 40 | 1.3 | 1.6 | 23000 | 13 | |
30 | 0.8 | 1.7 | 30000 | 12.5 |
2.1.1 Cutting speed analysis
The data in Table 3 shows that, with a laser power of 20KW as the test background, the cutting speeds of plates with thicknesses of 20mm and 30mm made of Q345, 45# steel, NM400, and T10 were compared, as shown in Figure 3.
With the same laser power, plate thickness, and oxygen as the auxiliary gas, the T10 plate had the slowest cutting speed, while NM400 had the fastest. There was no significant difference between the cutting speeds of Q345 and 45# steel.
It can be concluded that the carbon content in the cutting material has the most significant impact on the cutting speed. As the carbon content in the plate increases, the cutting speed of the plate with equal thickness decreases gradually. Also, as the content of rare elements (such as Cr, Ni) in the plate increases, the cutting speed decreases gradually.
Fig. 3-20KW Cutting Speed Comparison
2.1.2 Auxiliary air pressure analysis
Laser cutting carbon steel with oxygen as the auxiliary gas works by using the energy generated by the laser light source and the oxidation reaction during the cutting process.
It is evident that the oxygen pressure has a significant effect on various types of plates.
Table 4 in the process data of cutting 20mm and 30mm Q345, 45# steel, and T10 with a 20KW laser, as shown in Table 3, reveals that for different types of plates with the same thickness, the auxiliary gas pressure increases as the carbon content in the plates increases, for optimal cutting results.
Fig. 4-20KW Auxiliary Air Pressure Comparison Diagram
2.1.3 Cutting focus analysis
The data from the previous test shows that when cutting Q235, Q345, 45# steel, and T10 with the same thickness and using oxygen as the cutting auxiliary gas, 45# steel and T10 steel contain more carbon than Q235 and Q345.
During the cutting process, a large number of carbon dioxide pores form on the surface, causing a rough surface.
The cutting effect remains unchanged when the cutting focus changes within ±1, so the focus can be reduced to improve the cutting speed. However, the cutting effect of Q235 and Q345 is sensitive to the cutting focus, so they do not have this advantage.
The table below displays the results of cutting different types and thicknesses of plates using various auxiliary gases and cutting powers.
As shown in Table 4, it is evident that the cutting effects of different types of plates with the same thickness were compared by using the same cutting power.
The results indicated a significant difference in surface roughness among the samples cut from different materials, with the Q345B sample exhibiting the best cutting effect. The surface oxide skin was thin, and the surface roughness was minimal.
On the other hand, the oxide skin on the cutting surface of the NM400 sample showed obvious stratification. The upper side of the cutting surface was smooth, while the lower side had a thicker oxide skin, leading to a higher surface roughness for the sample.
The cutting of the 45# sample was rough, with an obvious protrusion of the oxide skin on the lower side.
The T10 sample had the poorest cutting effect, with a rough surface, numerous pores, and a noticeable oxide skin on the lower side.
In comparison, the Q345B, NM400, and 45# cutting surfaces had better oxide skin surface roughness than the T10 plate.
Table 4 Experimental Effect Table
2.2.1 Material melting point analysis
This experiment tested four types of materials: Q235, Q345B, NM400, and 45# carbon steel plates. Their carbon content is 0.22%, 0.20%, 0.25%, and 0.47% respectively.
By examining the iron carbon phase diagram in Figure 5, it can be observed that the melting point temperature of these four materials is approximately 1500°C.
Laser cutting of carbon steel utilizes the laser as a preheating heat source and oxygen as the auxiliary gas. This creates a highly exothermic oxidation reaction with the materials, releasing a significant amount of oxidation energy (as shown in the following formula).
Fe+O→FeO+heat(257.58kJ/mol)2Fe+1.5O2→Fe2O3+heat(826.72kJ/mol)
It has been established that the temperature at the processing location of the plate has exceeded 1726.85°C due to the energy released from the laser and the oxidation process during laser processing. This temperature is significantly higher than the melting points of the materials Q235, Q345B, NM400, and 45#.
Based on this analysis, it can be concluded that the melting points of these materials have limited impact on the effect of the oxide scale on the surface after cutting.
Fig. 5-Fe-C Phase Diagram
2.2.2 Chemical composition analysis of materials
The chemical composition of the different steel plates used in this experiment was determined using a spectrum analyzer. The results are shown in Table 5.
Table 5 Chemical Element Analysis
Chemical element/%/plate type | Q345 | Q235 | Q460 | NM400 | Q690 | 45# | T10 |
C | 0.2 | 0.22 | 0.2 | 25 | 0.18 | 47 | 1 |
Mn | 1.7 | 0.65 | 1.8 | 1.6 | 2 | 0.65 | 0.4 |
Si | 0.5 | 30 | 0.6 | 0.7 | 0.6 | 27 | 0.35 |
S | 0.035 | 0.05 | 0.03 | 0.01 | 0.02 | / | 0.02 |
P | 0.035 | 0.045 | 0.03 | 0.025 | 0.025 | / | 0.03 |
Cr | 30 | 0.3 | 0.3 | 14 | 1 | 0.25 | 0.25 |
Ni | 0.5 | 0.3 | 0.8 | 1 | 0.8 | 0.3 | 0.2 |
Cu | / | 0.3 | / | / | 25 | 0.3 | |
Mo | 0.1 | / | / | 0.5 | 0.3 | / | / |
Nb | 0.07 | / | 0.11 | / | 0.11 | / | / |
V | 0.15 | / | 0.2 | / | 0.12 | / | / |
Ti | 200 | / | 0.2 | / | / | / | / |
AI | 0.015 | / | / | / | / | / | / |
B | / | / | / | 0.004 | 0.004 | / | / |
1) Mn element content analysis
According to Table 5, which compares the Q235 and Q345B elements, both materials are classified as low-carbon steel. The content of other elements in the materials is not significantly different, except for the manganese content, which is 0.65% for Q235 and 1.70% for Q345B. This difference in manganese content serves as a benchmark for exploring the relationship between laser cutting quality and manganese content in the material.
The cutting surface effects of the two materials are shown in Figure 6. The results show that the surface is clean and bright, with similar surface roughness, and the experimental parameters were kept constant.
Based on these findings, it can be concluded that the Mn element has a slight impact on the laser cutting effect of conventional low-carbon steel.
Q235-20kw-20mm
Q345B-20kw-20mm
Fig. 6
2) S element content analysis
The data provided in the table shows that the maximum difference in the sulfur (S) element content among the sheets is only 0.05%. This information is not sufficient to determine the impact of S element content on cutting quality.
Further analysis of the data reveals that when the manganese (Mn) and sulfur (S) content in the plate are around 0.5% and 0.25% respectively, the slag at the bottom of the cutting surface increases with the increase in plate thickness, leading to a gradual decrease in cutting quality.
Table 6 Comparison of S and Mn Elements
Sheet/Element% | Q345 | Q235 | Q460 | NM400 | Q690 | 45# | T10 |
Mn | 1.7 | 0.65 | 1.8 | 1.6 | 2.0 | 0.65 | 0.4 |
S | 0.035 | 0.05 | 0.03 | 0.01 | 0.02 | 0.02 |
3) Si element content analysis
It has been observed that when the silicon (Si) element content in the metal plate is below 0.25%, the cutting speed of carbon steel plate with a Si content higher than 0.25% is slower by more than 20% compared to that of carbon steel plate with a Si content lower than 0.25%. Additionally, a substantial amount of slag will be produced at the bottom of the plate.
4) Content analysis of element C
When comparing the element content of Q235, 45#, and T10, it is found that Q235 is classified as low-carbon steel, 45# is medium-carbon steel, and T10 is high-carbon steel.
Examining the element table, it can be seen that the only significant differences are between carbon (C) and manganese (Mn).
Under high temperature and with sufficient oxygen as an auxiliary gas, carbon reacts with oxygen in the following manner:
C+O2→CO2(g)(393.5KJ/mol)
Theoretical analysis shows that as the carbon content of the material increases, the amount of carbon dioxide gas produced by the oxidation reaction will also increase in the presence of oxygen as an auxiliary gas, leading to an increase in the number of pores on the material’s cutting surface.
Figure 4 illustrates that as the internal carbon content of Q235, 45# steel, and T10 increases, the number of pores on the cutting surface also increases correspondingly.
Fig. 7 – Comparison Chart of Carbon Content of Materials
When comparing Q235 and Q345B materials initially, it was found that the manganese (Mn) element content has a minimal impact on the actual cutting effect and can be disregarded.
Figure 8 displays the actual cutting effect of the three materials with the same thickness. The results show that the surface of Q235 is bright with low roughness, the surface of 45# is rough with a significantly thicker oxide skin at the bottom, and the surface of T10 is the roughest with the thickest oxide skin.
From the actual test results, it can be concluded that the carbon content in the material has a noticeable impact on the cutting effect. As the carbon content increases, the number of pores on the cutting surface increases, the thickness of the surface oxide skin becomes thicker, and the surface roughness becomes greater.
Fig. 8-Q235-30kw-40mm (left), 45 # – 30kw-40mm (middle), T10-30kw (right)
5) Ni element content analysis
Table 7 displays the types and contents of chemical elements within Q235 and Q460 materials. The difference in nickel (Ni) element content between the two materials is evident.
Consequently, cutting tests were carried out on plates of the same thickness for both materials. The results of the actual cutting quality are shown in Figure 10.
There is no noticeable difference in the surface streaks, oxide skin thickness, and surface roughness.
Based on these results, it can be concluded that in conventional low-carbon steel, the nickel content has no significant impact on the cutting quality of high-power lasers.
Fig. 9 – Comparison Chart of Nickel Content in Materials
Table 7 Comparison of Ni Elements
Chemical element/% | Plate type | Q235 | Q460 |
C | 0.22 | 0.2 | |
Mn | 0.65 | 1.8 | |
Si | 0.3 | 0.6 | |
S | 0.05 | 0.03 | |
P | 0.045 | 0.03 | |
Cr | 0.3 | 0.3 | |
Ni | 0.3 | 0.8 | |
Cu | 0.3 | / | |
Mo | / | / | |
Nb | / | 0.11 | |
V | / | 0.2 | |
Ti | / | 0.2 | |
AI | / | / | |
B | / | / |
Q460-20mm-20KW
Q235-20mm-20KW
Fig. 10
6) Cr element content analysis
When comparing the content of elements in the plate, it is observed that the chromium (Cr) element content in materials NM400 and Q690 is significantly higher than in other materials, as illustrated in Figure 4.2-5.
Fig. 11 Comparison Chart of Chromium Content in Materials
During the laser cutting process, most elements in the plate will oxidize with the auxiliary gas, oxygen, and release a large amount of heat when the laser releases heat. This results in the formation of a significant heat-affected zone on the surface of the plate.
In this heat-affected zone, the chromium (Cr) in the plate will oxidize with oxygen and produce dense Cr2O3 and other oxides, which will increase with the local temperature. The oxide gradually grows and forms a cluster-like granular structure, as shown in Figure 12.
Over time, a Cr2O3 oxide skin with high surface stress and less prone to cracking forms on the metal cutting surface, which prevents the oxidation reaction between elements beneath the Cr2O3 oxidation and O2 (as shown in Figure 13). This results in significantly poor surface roughness on the undersides of the NM400 and Q690 cutting surfaces (as seen in Figure 14).
It can be concluded that the cutting effect worsens with the increase in Cr content in the material and the oxide skin at the bottom of the sample becomes thicker.
Fig. 12 – Phase diagram of clustered particles
Fig. 13 – Analysis Diagram of Laser Cutting Surface Oxide Layer
Cutting effect display 20mm NM400
Cutting effect display 20mm Q690
Fig. 14
It is understood that the quality of laser cutting is related to the heat-affected zone on the surface of the plate being cut. When the heat-affected zone is not controlled, it can cause distortions, cracks, brittleness, etc. on the surface of the cut plate.
According to the data comparison in Figure 15, it is known that the laser cutting power is the primary factor affecting the width of the cutting slit, and the cutting speed is the main factor affecting the streaks and roughness of the cutting surface.
Therefore, in laser cutting, it is recommended to adjust the process parameters as much as possible to minimize the area of the heat-affected zone on the plate surface, in order to reduce deformation and enrichment of components.
Fig. 15 – Influence of power and speed on kerf and cutting surface
During the actual testing process, the cutting process parameters were optimized to ensure smooth cutting surfaces and free-falling of samples of different types and thicknesses.
Under the same cutting power, there is no substantial difference in the width of slits among different types and with the same thickness.
As a result, the heat-affected zone area of materials with the same thickness is similar under the same power, having only a minor impact on the actual surface roughness and can be disregarded.
The factors that influence the cutting quality of carbon steel through oxygen cutting include the composition of alloys, material microstructure, thermal conductivity, melting point, and boiling point.
Metals with high carbon content typically have high melting points, making them difficult to melt, leading to an increase in the cutting and piercing time.
This results in a wider kerf and an expanded surface heat-affected zone, causing unstable cutting quality.
Related reading: Things You Should Know About Laser Cut Kerf
Furthermore, a high content of alloy composition increases the viscosity of liquid metal and raises the ratio of spatter and slag, imposing higher demands on the adjustment of laser power and air blowing pressure during processing.
Related reading: How to Select the Power of Fiber Laser Cutting Machine?
The above tests show that when oxygen is used as the auxiliary gas, the cutting surface effect worsens and the surface roughness increases significantly as the content of C and Cr elements in the material increases. On the other hand, when air is used as the auxiliary gas, the cutting effect remains largely unchanged under the same thickness and power.
To ensure the quality and efficiency of cutting, the recommended types of auxiliary gas for different cutting powers and materials are listed in the following table:
Under the same laser power, as the carbon content increases, the cutting speed decreases gradually, while the surface of the sample becomes rougher, the oxide skin becomes thicker, and the overall effect deteriorates, leading to a reduction in the thickness limit of laser cutting plates.
With increasing chromium content, the oxide skin at the bottom of the sample surface accumulates and thickens noticeably, causing the cutting surface to become rough from top to bottom.
When the silicon content in the material exceeds 0.25%, the cutting speed decreases significantly with increasing silicon content, and slag appears at the bottom of the cutting sample.
The nickel content has little effect on the quality of high power laser cutting.
When the content of manganese and sulfur in the material is 0.5% and 0.04% respectively, the slag at the bottom of the cutting increases gradually as the plate thickness increases.