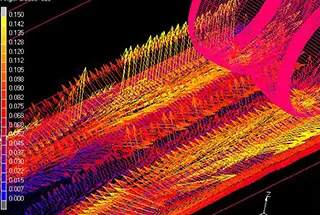
The mold undergoes a heat treatment process consisting of preheating, terminal heating, and surface hardening.
Heat treatment defects refer to various problems that occur during the final stage of the mold’s heat treatment or in subsequent processes and usage. These defects may include quenching cracks, poor dimensional stability, insufficient hardness, cracks from electromachining, grinding cracks, and early mold failure.
A more in-depth analysis is provided below.
The causes of quenching cracks and preventive measures are as follows:
Preventive Measures: Check and improve the design, including rounded corners, hole placement, and cross-section transitions.
Preventive Measures: Maintain and proofread the temperature control system, adjust the process temperature, and add iron between the workpiece and the furnace floor.
Preventive Measures: Use controlled atmosphere heating, salt bath heating, vacuum furnace, box furnace with box protection, or apply anti-oxidation coating, and increase the machining allowance by 2-3mm.
Preventive Measures: Understand the cooling characteristics of the quenching medium or tempering treatment and select the appropriate coolant.
Preventive Measures: Use the correct forging process and implement a reasonable preheat treatment system.
The reasons and precautions for insufficient hardness are as follows:
Preventive Measures: Correct the process temperature and overhaul and check the temperature control system. When installing the furnace, workpieces should be spaced evenly and not stacked or bundled for cooling.
Preventive Measures: Correct the process temperature and overhaul and check the temperature control system.
Preventive Measures: Correct the process temperature and overhaul and check the temperature control system. Enter the furnace at a temperature no higher than the set temperature.
Preventive Measures: Quickly enter the tank from the oven, understand the cooling characteristics of the quenching medium, add or cool the quenching medium if necessary, strengthen the stirring of the coolant, and remove at a temperature of Ms + 50°C.
Preventive Measures: Use controlled atmosphere and salt bath heating, vacuum furnace and box furnace with box protection or anti-oxidation coating, and increase the machining allowance by 2-3mm.
In the field of mechanical manufacturing, the occurrence of deformation during heat treatment is considered absolute, while the absence of deformation is relative. In other words, it all depends on the size. This is mainly due to the surface relief effect caused by martensite transformation during heat treatment.
Preventing deformation (changes in dimensions and shape) during heat treatment is a challenging task and often requires experience to solve. This is because various factors, such as the type of steel, the shape of the mold, the improper distribution of carbides, and the method of forging and heat treatment, can all contribute to or worsen the problem.
Additionally, changes in any of the various conditions during heat treatment can greatly impact the degree of deformation in the steel piece.
For a long time, solving the issue of heat treatment deformation was mainly done through experience and heuristics. However, it is crucial to have a thorough understanding of the relationship between mold steel forging, module orientation, mold shape, heat treatment method, and heat treatment deformation. This understanding can be gained by analyzing the accumulated data and establishing archives of heat treatment deformation.
Decarburization is a phenomenon and reaction in which carbon on the surface layer of steel is completely or partially lost due to the effect of the surrounding atmosphere during heating or insulation.
The decarburization of steel parts can result in insufficient hardness, quench cracking, heat treatment deformation, and chemical heat treatment defects. Additionally, it can significantly impact fatigue strength, wear resistance, and mold performance.
In mold manufacturing, electrical discharge machining (EDM) is becoming an increasingly common processing method. However, with its widespread use, the defects caused by EDM have also increased.
EDM is a machining method that melts the surface of a mold using the high temperature generated by electric discharge. This process forms a white EDM deteriorative layer on the machining surface and generates a tensile stress of about 800 MPa. As a result, deformation or cracks may occur during the electrical processing of the mold.
Therefore, when using EDM molds, it’s crucial to understand the impact of EDM on the mold steel and take preventive measures to avoid defects:
The insufficient toughness can be attributed to either excessively high quenching temperature and prolonged holding time, leading to grain coarsening, or failing to avoid tempering in the brittle zone.
The presence of a large amount of retained austenite in the workpiece can result in structural stress and cracking of the workpiece when the tempering transformation occurs during grinding heat. To prevent this, two preventive measures can be taken: performing a cryogenic treatment after quenching or repeating the tempering process (typically 2-3 times for low-alloy tool steels in cold working) to minimize the amount of retained austenite.
The mold design primarily depends on its intended use, and its structure may not always be completely rational and symmetric.
This requires designers to adopt effective measures during the mold design process. Without compromising the mold’s performance, they should pay attention to the manufacturability, structural rationality, and geometric symmetry.
(1) Avoid sharp corners and sections with large thickness differences.
Sections with drastic thickness differences, thin edges, and sharp corners should be avoided.
Smooth transitions should be employed at the junctions of thick and thin sections in the mold. This can effectively reduce the temperature differences across the mold cross-section, minimizing thermal stress.
It can also reduce the time disparity of structural transformations across the section, thus reducing structural stress. Figure 1 illustrates the use of transition fillets and cones in mold design.
(2) Incorporation of Additional Process Holes
For those molds that are genuinely challenging to ensure uniform and symmetrical cross-sections, one should, without compromising their functionality, convert blind holes into through holes or suitably integrate additional process holes.
Figure 3a illustrates a type of narrow cavity die, which upon quenching, undergoes deformation as depicted by the dotted lines. If two process holes are added during the design phase (as shown in Figure 3b), this reduces the temperature difference across the section during quenching, thereby lowering the thermal stress and significantly improving the deformation situation.
Figure 4 also demonstrates an instance of adding process holes or changing blind holes to through holes, which can reduce the increased susceptibility to cracking caused by uneven thickness.
(3) Utilize enclosed and symmetrical structures as much as possible
When the mold shape is open or asymmetrical, the stress distribution after quenching is uneven, which easily leads to deformation. Therefore, for generally deformable groove molds, it is advisable to leave ribs before quenching and cut them off after quenching.
As shown in Figure 5, the grooved workpiece originally deformed at point R after quenching. By adding ribs (the shaded part in Figure 5), quenching deformation can be effectively prevented.
(4) Implementing a Composite Structure
For complexly shaped, large-scale concave molds exceeding 400mm in size, as well as thin, elongated convex molds, it is ideal to employ a composite structure.
This approach simplifies complexity, reduces size, and transforms the interior surfaces of the mold into exterior ones. Not only does this facilitate thermal processing, but it also effectively minimizes deformation and cracking.
When designing a composite structure, the decomposition should generally follow these principles, provided they do not affect the precision of the fit:
(1) Adjust the thickness to ensure a uniform cross-section after decomposition, especially for molds with stark differences in their initial cross-sections.
(2) Decompose areas prone to stress concentration to distribute the stress and prevent cracking.
(3) Incorporate aligned process holes to maintain structural symmetry.
(4) Facilitate thermal processing and ease of assembly.
(5) Above all, usability must be assured.
Figure 6 illustrates a large concave die. Opting for a monolithic structure makes heat treatment challenging and results in inconsistent shrinkage across the die cavity after quenching.
This can even lead to uneven blade edges and planar distortion, which are difficult to remedy in subsequent processing. Hence, a modular structure can be used. As indicated by the dashed lines in Figure 6, the structure is divided into four parts.
After heat treatment, these parts are reassembled, ground, and fitted together. This not only simplifies the heat treatment process but also solves the deformation problem.
Heat treatment deformation and cracking are closely related to the steel used and its quality. Therefore, the material should be selected based on the performance requirements of the die.
Factors such as die precision, structure and size, as well as the nature, quantity, and processing methods of the workpiece should be considered.
Generally, if there are no deformation and precision requirements for the die, carbon tool steel can be used to reduce costs. For components prone to deformation and cracking, high-strength alloy tool steel with slower critical quenching cooling speeds can be chosen.
Figure 7 shows a die for an electronic component. Originally, T10A steel was used, and the water-quenching and oil-cooling process led to significant deformation and susceptibility to cracking.
Moreover, alkaline bath quenching made it difficult to harden the die cavity. Now, 9Mn2V steel or CrWMn steel is used, meeting the requirements for quenching hardness and deformation.
It is evident that when molds made of carbon steel fail to meet deformation requirements, replacing it with alloy steel such as 9Mn2V or CrWMn can solve the deformation and cracking issues.
Despite the slightly higher material cost, it’s still cost-effective in the grand scheme of things.
Simultaneously, along with making the right material choice, it is crucial to enhance inspection and management of raw materials to prevent mold heat treatment cracks due to raw material defects.
The rational formulation of technical conditions, including hardness requirements, is a crucial path to prevent quenching deformation and cracking.
If localized hardening or surface hardening can meet the usage requirements, try to avoid quenching the entire piece.
For wholly quenched molds, where localized requirements can be relaxed, uniformity should not be strictly pursued.
For high-cost or complex structure molds, when heat treatment cannot meet the technical requirements, the conditions should be altered, appropriately slackening those demands that have little impact on the lifespan to avoid scrapping due to multiple reworks.
The steel type chosen should not have its maximum achievable hardness set as the design’s technical condition.
This is because the maximum hardness is often measured with limited-size samples, which can significantly differ from the hardness attainable by larger, actual-size molds.
Since pursuing maximum hardness often requires increasing the quenching cooling speed, leading to a higher tendency for quenching deformation and cracking, using a higher hardness as a technical condition can pose certain challenges even for smaller-sized molds during heat treatment.
In summary, the designer should formulate feasible technical conditions based on usage performance and the selected steel type.
Additionally, when setting hardness requirements for the selected steel type, one should avoid the hardness range that may cause temper brittleness.
The correct management of the relationship between mechanical processing and heat treatment, and the rational arrangement of the technological process, enabling a close coordination between cold and hot working, are effective measures to reduce mold heat treatment deformation.
In some cases, the deformation of a mold cannot be resolved solely from the perspective of heat treatment. However, shifting the mindset and addressing the entire technological process often yields unexpected results.
Figure 8 shows a half-round mold, which due to its asymmetrical shape, undergoes significant twisting deformation during quenching.
If it is machined into a full ring before quenching, and then cut into two pieces with a saw blade grinding wheel after heat treatment, not only can costs be reduced, but deformation can also be minimized.
Distortion is inevitable during processing.
If its characteristics can be understood, and adequate processing allowances can be reasonably reserved, not only can the heat treatment operation be simplified, but subsequent mechanical processing, especially grinding work, can be reduced.
Figure 9 shows a forming mold of 45# steel. After heat treatment, the inner hole tends to expand, so a negative tolerance should be reserved in advance during mechanical processing to meet the design requirements after heat treatment.
For molds where the size and direction of deformation cannot be anticipated in advance, a trial quenching can be performed before the mold cavity has been machined to design dimensions.
Based on its deformation characteristics, the corresponding mechanical processing allowance can be reserved.
For precision molds, the stress generated by cutting or grinding processes can cause deformation and cracking.
Therefore, incorporating stress relief annealing or aging treatment in the processing flow can significantly reduce deformation and prevent cracking.
For instance, for slender shaft-type and complex-shaped molds, performing a stress relief annealing after rough machining to eliminate the stress from cutting is highly effective in reducing quenching deformation.
Similarly, for some molds that require precision grinding, an aging treatment can be scheduled after heat treatment and rough grinding to eliminate the stress from grinding, stabilize dimensions, and prevent deformation and cracking.
Band-like structures and compositional segregation in steel often lead to uneven deformation of molds. The state of the matrix organization before quenching can also affect the volume difference of the mold before and after quenching.
Under certain conditions, the quality of the original structure in the steel becomes a major factor affecting heat treatment deformation.
In order to minimize quenching deformation, in addition to taking effective measures during the quenching process, the structure inside the steel before quenching should also be properly controlled.
Experience proves that rational forging is key to minimizing heat treatment deformation and ensuring that the mold has a longer lifespan. This is especially important for alloy steels (such as CrWMn, Cr12, and Cr12MoV steels).
The premise for these types of steels to achieve low deformation is through sufficient forging, enabling the degree of carbide segregation inside the steel to be minimized.
Therefore, the forging process must be correctly controlled in the following five aspects:
(1) Forging Method: The shaping process requires multiple forging steps, typically no fewer than three for high-alloy steel, to ensure the carbides are fractured and evenly distributed.
(2) Forging Ratio: A certain forging ratio is necessary. For instance, the total forging ratio for high-alloy steel is generally between 8-10.
(3) Heating Speed: Gradually heat up to approximately 800°C, then slowly increase the temperature to 1100-1150°C. During the heating process, the workpiece should be regularly flipped to ensure uniform heating and thorough penetration.
(4) Final Forging Temperature Control: If the final forging temperature is too high, the grain size tends to increase, resulting in poorer performance. On the other hand, if the final forging temperature is too low, the material becomes less ductile, prone to band-like structures, and may easily fracture.
The deformation and cracking of molds are not only associated with the stress generated during the quenching process but also with the original structure and residual internal stress prior to quenching. Therefore, it is essential to implement the necessary pre-heat treatment for the mold blanks.
Typically, smaller molds made of T7 and T8 steel tend to expand in volume during quenching. If tempered beforehand, a larger tempered sorbite structure than the original volume can be achieved, reducing the deformation during quenching.
On the other hand, larger molds manufactured with high-carbon steel like T10 and T12 steel tend to contract in volume when quenched. In this case, spheroidizing annealing should be adopted, which can yield better results than tempering.
For low-alloy tool steels, arranging a tempering process after mechanical machining can distribute the alloy carbides evenly, significantly improving the structure and mitigating the adverse effects of the forging and original structures.
The tempering process results in evenly distributed carbides and a fine-grained sorbite structure, increasing the comparative volume of the original structure.
This not only enhances the mechanical properties of the steel but also aids in minimizing deformation. For high-alloy tool steels (such as high-chromium steel) molds, different degrees of contraction may occur during quenching after tempering.
Therefore, replacing the high-temperature tempering with annealing during the tempering process can yield better results after quenching.
Alloy structural steel can achieve higher hardness through pre-tempering treatment, which also minimizes volume changes during quenching, reducing potential deformations and cracking.
The use of low-temperature annealing to alleviate the cold working stress in molds is simpler than tempering, with a shorter cycle, less oxidation, and applicability to various materials using the same process.
In order to eliminate the network carbides caused by poor forging and increase the depth of the hardened layer, normalizing treatment can be applied.
In summary, all types of pre-heating treatment should be conducted according to the mold’s expansion and contraction patterns, adjusting the initial structure and eliminating machining stress to reduce deformation and cracking.
To minimize and prevent workpiece quenching distortion, not only does it require a rational design of the workpiece, selection of materials, formulation of heat treatment technical requirements, and correct thermal processing (casting, forging, welding) and pre-heat treatment of workpiece blanks, but it is also essential to pay attention to the following issues in heat treatment:
(1) Rational Selection of Heating Temperature
To ensure hardening, the quenching temperature should generally be as low as possible. However, for certain high-carbon alloy steel molds (such as CrWMn, Cr12Mo steel), quenching distortion can be controlled by appropriately raising the quenching temperature to lower the Ms point, increasing the amount of residual austenite.
Additionally, for thicker high-carbon steel molds, the quenching temperature can also be raised slightly to prevent the occurrence of quenching cracks.
For molds that are prone to distortion and cracking, stress relief annealing should be performed before quenching.
(2) Rational Heating Process
Uniform heating should be achieved as much as possible to minimize the thermal stress during heating.
For large cross-section, complexly shaped, high-alloy steel molds with high distortion requirements, preheating or restricted heating rates should typically be applied.
(3) Correct Selection of Cooling Method and Medium
Pre-cooling quenching, step quenching, and step cooling methods should be selected as much as possible.
Pre-cooling quenching has a good effect on reducing distortion in long, thin, or thin molds, and to some extent, it can reduce distortion in molds with significant thickness variations.
For complex shapes or molds with significant sectional differences, step quenching is preferable. For example, using step quenching at 580-620°C for high-speed steel essentially avoids quenching distortion and cracking.
(4) Correct Mastery of Quenching Operation Methods
The correct choice of the way the workpiece is immersed in the medium should ensure the most uniform cooling of the mold and entry into the cooling medium along the path of least resistance, with the slowest cooling side facing the direction of the liquid movement.
When the mold cools to below the Ms point, the movement should stop. For example, molds with uneven thickness should be immersed with the thicker part first; workpieces with significant section changes can reduce heat treatment deformation by increasing process holes, reserving reinforcement ribs, plugging holes with asbestos, etc.
For workpieces with concave surfaces or through holes, the concave side and holes should be immersed upwards to expel the bubbles inside the through holes.
Heat treatment is an indispensable manufacturing process in mold production, significantly impacting both the quality and cost of the mold, and serves as a crucial measure to enhance its lifespan. Deformation and cracking are two major challenges during mold heat treatment.
The causes behind these issues are complex, but by understanding their patterns, conducting thorough analysis and research, and addressing the problems accurately, it is possible to reduce mold deformation and control cracking effectively.
As the founder of MachineMFG, I have dedicated over a decade of my career to the metalworking industry. My extensive experience has allowed me to become an expert in the fields of sheet metal fabrication, machining, mechanical engineering, and machine tools for metals. I am constantly thinking, reading, and writing about these subjects, constantly striving to stay at the forefront of my field. Let my knowledge and expertise be an asset to your business.