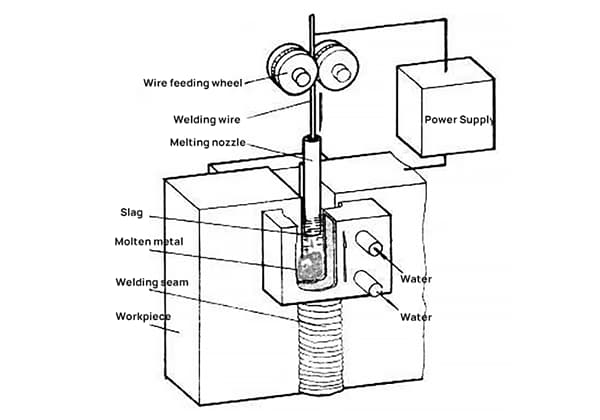
Welding fumes pose a significant hazard to both the environment and worker health, containing toxic gases and fine particles that can lead to serious respiratory and systemic illnesses. This article explores the formation, dangers, and current control methods of welding fumes, emphasizing the urgent need for effective solutions in industrial settings. Readers will gain insights into the mechanisms of fume generation, health risks, and practical strategies to improve air quality and safety in welding operations.
Welding is a widely used basic process in various industrial manufacturing fields. However, it generates harmful byproducts such as arc light, electromagnetic radiation, toxic gas, and smoke particles. These byproducts not only pollute the environment but also pose a great risk to the health of employees.
Among these hazards, welding fume is the most complex and challenging to control in welding production. Therefore, conducting research on welding fume control is essential to improve the welding production environment and protect employee health.
This article provides a summary of the formation mechanism, hazards, and treatment measures of welding fume. It also analyzes the challenges encountered in treating welding fume in engineering applications and identifies the development direction of welding fume treatment.
Related reading: The Ultimate Guide to Welding
As a fundamental process of modern manufacturing, welding technology has evolved from traditional single-connection methods to a multi-disciplinary hot forming technology that integrates electricity, machinery, materials, and computers. It plays an irreplaceable role in various fields such as engineering machinery, water conservancy and hydropower, shipbuilding, transportation, military equipment, and others.
However, the welding process emits arc radiation, high temperature, noise, welding fumes, and toxic gases that not only pollute the environment but also endanger employees’ health.
While masks and earplugs offer good protection against arc light, high temperature, and noise, they are not as effective against welding fumes, which are primary carcinogens.
Welding fumes primarily contain toxic gases and soot particles. The soot particles may cause asthma, bronchitis, pneumonia, pulmonary edema, acute poisoning, nervous system diseases, and even pneumoconiosis, metal fume heat, respiratory function changes, cancer, and other diseases.
Toxic gases like asphyxiating gas CO, irritating gases like ozone, fluoride, chloride, sulfur dioxide, and nerve toxic gases like nitrogen oxide, phosgene can cause employees to have headaches, dizziness, coughs, expectoration, chest pain, tinnitus, tension, and anxiety.
The welding environment’s poor quality due to welding smoke and other associated hazards has resulted in a decline in the number of people willing to engage in welding work year by year. This has become one of the prominent problems restricting the welding industry’s healthy development.
In recent years, with the substantial increase in welding manufacturing workload and the popularity of efficient welding methods, such as flux-cored wire and other high dust-producing processes and materials, the occupational problems caused by welding fumes have become increasingly prominent.
In the early days, China’s allowable concentration of smoke and dust in workshop air was 6mg/m3.
Currently, the China Welding Association has decreased the allowable concentration of smoke and dust to 4mg/m3 and has set clear requirements for the quantity of dust generated by welding materials. In light of the risks associated with welding fumes, the American Welding Society has also developed a ventilation manual to reduce the concentration of welding fumes in workshops.
At the turn of the century, Japan established relevant standards to restrict the concentration of smoke and dust in welding workshops and actively promoted the research and development of new welding materials.
However, in actual production, particularly in areas with high welding intensity and relatively enclosed spaces, such as shipyard workshops, the soot concentration can reach 9-18 mg/m3 and even 38-312 mg/m3 in cabin sectional confined spaces, which is well above the minimum concentration of soot required by the standards and is extremely detrimental to human health.
It is clear that reducing hazardous substances in smoke and dust and enhancing the working environment for welding professionals has become an urgent issue that needs to be resolved in the welding industry.
At present, the treatment of welding fume at home and abroad is mainly carried out in the following three directions:
(1) Strengthen Personal Protection; (2) Optimize Welding Process and Materials; (3) Ensure Adequate Ventilation and Smoke Exhaust.
This article analyzes the generation mechanism of welding fumes and compares the advantages and disadvantages of current mainstream fume control measures. It proposes a new concept of welding fume control through intelligent manufacturing, which provides a useful reference for improving welding fume control.
Due to the physical and chemical hazards of welding fumes to employees, it is crucial to protect their health and maintain air quality in the welding operation environment. This can be achieved by understanding the generation mechanism and influencing factors of welding fumes, exploring the generation, growth, and polymerization process of welding fumes, and controlling and protecting fumes at the source.
Furthermore, this approach lays a theoretical foundation for the industry’s admission standards for welding fumes.
Early research suggested that the generation mechanism of welding fumes was a process involving overheating, evaporation, oxidation, and condensation, as illustrated in Figure 1.
In the welding process, the temperature at the arc center is high, causing the evaporation of both liquid metal and non-metallic substances, which in turn generates high-temperature steam and maintains a certain particle concentration.
As the high-temperature steam reaches the low-temperature area at the edge of the arc, it rapidly oxidizes and condenses, resulting in the formation of “primary particles.”
These primary particles are generally spherical and have a diameter of 0.01-0.4 μm, with a majority being 0.1 μm.
Due to the static electricity and magnetism of the primary particles themselves, they will polymerize and form “secondary particles” as the temperature decreases, which then diffuse in a specific manner.
Shi Yuxiang, from Wuhan Jiaotong University of Science and Technology, conducted an in-depth study on the formation mechanism of welding fumes. He proposed an aerosol mechanism for welding fumes, which aimed to explain the transformation process of steam and fume.
He suggested that the nucleation mechanism of welding aerosol particles near the arc is divided into homogeneous nucleation and heterogeneous nucleation.
The spectral distribution, morphology, composition, and structural characteristics of primary particles were systematically investigated both experimentally and theoretically through direct sampling electron microscopy and DMPS.
It was discovered that Fe3O4 crystals were mainly composed of particles of 0.01μm in scale, which originated from welding aerosols, while smoke particles of 0.1μm in scale had two types of crystal structures, spinel type and fluoride type, which were formed by the heterogeneous condensation mechanism of vapor particle transition.
Soot particles above 1μm in scale were primarily formed by the bubble particle transition mechanism. Additionally, a model of the welding arc particle nucleation zone is proposed, which is of great significance in analyzing the formation process of welding aerosol particles.
The soot particles generated during welding undergo growth in the diffusion process through aggregation and fusion.
During the fusion process, several primary particles fuse into a single large particle, where the total surface area of the latter is less than the sum of the surface areas of the primary particles, and no boundary exists between them.
In contrast, the aggregation process is comprised of dozens, or even hundreds, of primary particles that adhere to the surface, exhibiting distinct boundaries between particles.
Regardless of whether particle aggregation or fusion occurs, the particle size, shape, and concentration in the welding fume will undergo changes.
The welding process can produce a large amount of smoke and toxic gases that are harmful to human health.
A significant proportion of welding smoke consists of suspended particles that diffuse in the air, while another portion is dispersed as toxic gas.
The soot particles produced during welding primarily exist as metal oxides, which are complex in composition, highly viscous, and have high temperatures, with non-uniform particle sizes.
Typically, welders have a breathing capacity of about 20 L/min in welding production environments. Therefore, their breathing capacity for one year is approximately 2300m3.
In poor welding production environments, a worker may inhale 100g of particles per day and 2.5kg of harmful substances over 25 years of work.
Table 1 displays the common metal oxide particles produced during welding and their associated hazards.
Table 1 Hazards of particles in welded fume
Material | Source | Hazard |
Ferric oxide | From filler material and base metal | Iron pneumoconiosis or iron deposition disease caused by long-term inhalation |
Aluminum oxide | Welding process from aluminum base materials | Dust deposition in the lung causes pneumoconiosis |
Manganese oxide | Welding process from manganese containing welding materials | Irritating to the respiratory tract, causing pneumonia. Long term exposure will damage the nervous system |
Oxide | Basic electrode or coated wire | Irritating to gastric mucosa, causing bone damage |
Barium compound | Barium containing welding filler | Toxicity, causing potassium deficiency in human tissues |
Nickel oxide | Welding materials of pure nickel or nickel base alloy | Nasal mucosa damage and lung cancer, Class I carcinogen |
According to the particle size, welding fumes can cause varying degrees of harm to the human body.
The team led by Yang Lijun at Tianjin University conducted research on the particle size distribution of MIG welding fumes, analyzed the impact of welding parameters and droplet transfer on the fume particle size. The results showed that soot particles exhibited quasi-quantized distribution characteristics, with particle sizes mostly falling within the range of 0.1 to 1 μm, accounting for more than 85%, and particle sizes less than 0.1 μm accounting for about 10%. Additionally, welding processes, droplet transfer forms, and welding parameters all had certain effects on the size of soot particles. Specifically, decreasing the welding voltage led to a reduction in the size of soot particles.
Gomes JF et al. calculated that the particle size of welding fumes generated during the welding process was approximately 0.5 μm.
Research has shown that smoke particles with diameters of more than 10 μm in the air are deposited in the nasopharynx, while those with diameters of less than 10 μm can be inhaled by the human body. Smoke particles with diameters of 2 to 10 μm can be discharged, but those with diameters of less than 0.5 μm will be deposited in the lungs and are difficult to remove.
Table 2 shows the residual amount of TiO2 with different particle sizes in rat lung tissue for several days (unit: μg). The smaller the particle size, the more penetrable it is and the more difficult it is to eliminate from the body. Furthermore, smoke particles will disperse into smaller primary particles in the human alveoli, exacerbating their harmful effects on the body.
Table 2 Content of different sizes of TiO2 in rat lung tissue (μg)
Time/day | TiO2-D(0.03μm) | TiO2-F(0.25μm) |
1 | 347.7±13.1 | 324.3±6.1 |
29 | 202.8±23.0 | 172.8±12.1 |
59 | 140.9±22.6 | 128.5±16.6 |
Lauryn M. F. et al. found that Fe2O3 is the only metal oxide that promotes lung cancer, and the trend of metal oxides causing lung inflammation is Fe2O3 > Cr2O3+CaCrO4 > NiO. Among them, the toxic effect of Fe2O3 on the lung is continuous, while the toxic effect of Cr2O3+CaCrO4 on the lung is acute.
Roth J. A. et al. found that prolonged exposure to welding fumes and excessive inhalation of manganese can have adverse effects on human health, including damage to the lungs, liver, kidneys, and central nervous system. Male workers are at a higher risk of infertility.
Long-term exposure to environments with manganese concentrations exceeding 1 mg/m3 can increase the risk of manganese poisoning, similar to Parkinson’s disease.
In addition to the many harmful smoke particles produced by welding, it also emits many harmful gases, including carbon monoxide, nitrogen oxides, ozone, phosgene, hydrogen fluoride, and other harmful components.
Table 3 lists the hazards of the harmful gases in some welding fumes to the human body.
Table 3 Harmful gases and hazards in welding fumes
Harmful gas | Produce | Hazard |
Carbon monoxide | The welding flux or shielding gas is produced by carbon dioxide combustion and decomposition. | Headache, dizziness, confusion, suffocation |
Nitric oxide | It is produced by the action of ultraviolet ray generated by electric arc on nitrogen in the air | Irritating eyes and respiratory tract, leading to pulmonary congestion |
Ozone | It is produced by the interaction of ultraviolet ray generated by arc and nitrogen in air | The respiratory tract feels dry, causing headache, fatigue, pulmonary congestion and pulmonary disease |
Phosgene | It is produced by decomposition of fluoride containing solvent, polytetrafluoroethylene, surface coating, etc. | Irritating respiratory tract, nose and eyes, toxic, leading to pulmonary edema. |
Hydrogen fluoride | Electrode coating and flux | Irritating eyes, nose, throat, lung congestion, bone changes |
Comprehensive measures should be taken to purify the welding working environment and protect employees’ health by reducing emissions from the source, strengthening protection, and promoting technological innovation. This will help to ensure that the concentration of harmful substances generated by welding remains within the allowable range.
Currently, there are several common treatment measures available, such as personal protection, optimizing the welding process and materials, and implementing ventilation and smoke exhaust systems.
Personal protection measures for welding fumes mainly involve wearing ventilation and dust removal masks, as well as other respiratory protection equipment, to reduce the harm caused by welding fumes to workers.
Figure 2 illustrates four filtering mechanisms of respirators for smoke and dust particles of various sizes.
(1) Gravity effect:
As air containing dust particles passes through the fiber layer of the filter material, the particles are displaced from the airflow direction by their own gravity and are deposited on the filter material.
Typically, dust particles larger than 1μm in size are filtered out effectively, while smaller particles can be ignored due to their minimal effect on gravity in comparison to gas flow rate and other factors.
(2) Interception effect:
The fibers within the filter material are irregularly stacked and intertwined with one another.
As high-speed smoke particles in the air come into contact with the fiber material, they bond to the surface of the fibers, resulting in effective particle interception.
(3) Inertia effect:
Smoke particles are deposited on the fiber surface due to the effect of the inertial force, as the air flow changes direction frequently while passing through the filter material, causing them to break away from the streamline. This phenomenon is particularly true for smoke particles with a particle size of 0.5~1.0μm, which are mainly intercepted by the inertial effect.
(4) Diffusion effect:
Particles with a diameter of less than 0.1 μm at room temperature mainly move through Brownian motion. The smaller the particles, the easier they are to remove.
Particles larger than 0.5 μm are mainly in inertial motion. The larger the particles, the easier they are to remove.
Particles between 0.1 μm and 0.5 μm have no obvious diffusion and inertia effects and are difficult to remove.
During welding, the size of smoke particles ranges from 10-3 to 102 μm in five orders of magnitude, with 0.1-0.5 μm particles being the most penetrating.
Currently, no respirator can achieve an ideal filtering effect on all smoke particles.
Personal protective equipment currently has a poor protective effect on toxic gas, and the prevention of toxic gas cannot be achieved solely through personal protection.
The optimization of welding processes and materials mainly involves controlling welding fumes by reducing the generation rate of fumes and the content of toxic substances within them.
There are numerous factors that affect the quantity of welding dust produced.
Currently, research on welding dust in domestic and international settings mainly focuses on two aspects:
The first is studying the influence of various welding methods and process parameters on the amount of dust generated, and the second is studying the impact of the composition of welding wire, coating, and shielding gas on the amount of dust produced.
2.1 Impact of welding process on dust emission
The amount of dust generated varies depending on the welding method used.
When the same process parameters are used, MIG welding produces a much higher dust generation rate than non-MIG welding. Conversely, the smoke generated by submerged arc welding is minimal.
Related reading: MIG vs TIG Welding
Table 4 displays the amount of dust generated by various welding methods under identical specifications.
In general, when using the same welding method, the amount of dust produced increases as the welding current and voltage rise.
Compared to DC welding, AC welding generates a greater amount of dust, but the quantity of dust diminishes as the welding speed increases.
Table 4 Dust generation rate of different welding methods
Welding process | Generation rate/(mg·min-1) |
FCAW | 900~1300 |
SMAW | 300~800 |
MIG/MAG | 200~700 |
GTAW | 3~7 |
SAW | 3~6 |
The generation of large amounts of dust from flux cored wire welding, shielded metal arc welding, and MIG welding has a serious impact on both welders and the environment, making it a major research focus both domestically and internationally.
Shi Qian and colleagues from Lanzhou University of Science and Technology conducted research on the amount of dust generated by self-shielded flux cored wire welding under different process parameters.
Their findings indicate that in small specification welding, the amount of dust generated significantly increased due to the increase in spatter during short circuit transition and slag column transition. In large specification welding, the evaporation rate of the droplet and base metal heated is accelerated due to the increase in heat input, resulting in an increase in the amount of dust generated. The droplet transfer mode had little effect on the amount of dust generated.
These results were also confirmed in Zhang Junqiang’s research on the generation mechanism of smoke and dust from self-shielded flux cored wire welding. The study found that the aggregate smoke and dust generated in the splash smoke and dust area and the droplet smoke and dust area greatly increased the total amount of smoke and dust.
Yamamoto et al. used CO2 as a shielding gas when welding with 26% flux-cored wire.
With an increase in welding current, the amount of welding dust gradually decreases.
The author has also developed an advanced pure carbon dioxide gas shielded arc welding process by using the pulse current method to control the droplet.
This method uses high current to melt the welding wire and then reduces the current during droplet transfer. This ensures that the droplet can be smoothly transferred to the molten pool with a constant length, resulting in regular formation and separation of metal droplets, and reducing the amount of dust generated by 50%.
Scotti studied the influence of arc length, droplet diameter, and short circuit current on the amount of dust generated by GMAW using a control variable method.
The results show that during short circuit transition, an increase in droplet diameter, short circuit current, and arc length leads to an increase in the amount of dust generated. Higher short circuit current makes metal evaporation on the surface of the liquid bridge more intense when the droplet enters the molten pool, increasing the amount of dust generated. When these factors work together, the increase in dust emission is more noticeable.
Bu Zhixiang of Hubei University of Technology and others conducted an orthogonal experiment with CO2 gas shielded welding of solid welding wire as the research object, and used welding current, welding voltage, and welding speed as the three experimental factors. They took the welding dust rate and amount as the experimental indicators.
Through variance analysis and range analysis of orthogonal test data, the results show that the main factors affecting the formation rate of welding fume are welding current and welding voltage, and welding speed has no significant effect on the formation rate of welding fume. When the welding voltage is 22-24 V, the welding current is 290-320 A, and the welding speed is 26 cm/min, the amount of welding dust is the lowest.
The amount of welding fume is not only related to the filler material, but also closely related to the composition of the shielding gas.
K. R. Carpenter et al. added O2 and CO2 to the protective gas of GMAW and found that adding 2% O2 into the binary Ar-CO2 mixture had no effect on the dust generation rate.
When O2 in the ternary mixture increases, the dust generation rate increases at the level of 5% CO2, but does not increase significantly at the level of 12% CO2.
The amount of dust generated can be controlled by adjusting the amount of CO2 added to the mixed gas, according to a study by Li Zhuoxin’s team from Beijing University of Technology on the Cr (Ⅵ) content in stainless steel welding fume.
Their results indicated that the mass fraction of Cr (Ⅵ) in the fume increased with stronger oxidation of the shielding gas during gas shielded welding. In addition, Cr (Ⅵ) increased with higher electric currents (150~250 A) during MAG welding, and the mass ratio of Cr (Ⅵ) in the short circuit transfer fume to total Cr was higher than that of the jet transfer fume during GMAW.
A report presented by Vishal Vats at the interim meeting of the Eighth Committee of the 2022 IIW pointed out that adding oxygen to GMAW protective gas would promote the formation of Cr3+ and Cr6+, as well as increase harmful elements like Mn, Fe, and Ni in the smoke.
These findings suggest that the amount of welding dust is influenced by welding process parameters, and selecting suitable parameters can reduce dust emissions and promote a healthier environment. However, there is a coupling effect between welding process and welding quality that may require sacrificing quality and efficiency to reduce smoke emissions, which presents limitations in practical applications.
The increasing use of efficient welding methods (double wire/multi wire welding, laser arc hybrid welding) in engineering further raises welding specification requirements and makes welding fume treatment more challenging.
2.2 Effect of welding materials on dust emission
During the welding process, metal oxides produced by welding materials at high temperatures mix with various carcinogens. If operators inhale these particles excessively, it can cause a range of diseases.
To mitigate these risks, the development of green welding materials can effectively control the harmful components of smoke and dust at the source.
The research on green welding materials at home and abroad mainly focuses on three aspects:
(1) By modifying the composition of the drug skin, it is possible to reduce the amount of dust generated by the material.
(2) To reduce the content of heavy metal elements in welding smoke and dust.
(3) Welding fumes should be treated by using dealloying welding materials.
The amount of dust generated during welding is influenced by the coating composition of the electrode, chemical composition of the powder, and the welding wire steel strip. The influencing factors are complex.
Fluorite and sodium silicate are major contributors to the generation of dust in electrode coating, and their reaction products account for more than 50% of the total amount of smoke and dust.
Materials containing K and Na increase the amount of dust generated, whereas silicon calcium alloy and magnesium powder can inhibit it.
Research by Jiang Jianmin and others at Beijing University of Technology found that reducing the content of iron powder in the flux core of the wire can decrease the amount of dust generated during welding by 33% to 47%.
According to a report by Mruczek MF, a foreign welding material manufacturer has developed a low manganese content flux cored wire that can effectively reduce the Mn content in welding fume. However, this may lead to poor mechanical properties of the weld.
North T H has found that adding Mn containing composite particles into the core can significantly reduce the Mn content in welding fume by preventing Mn oxidation and leaving more Mn in the weld.
Dennis J H et al. added active elements (Zn, Al, Mg) to the flux cored wire, which can significantly reduce the Cr6+ content in welding fume by allowing the active elements to preferentially oxidize. However, adding Zn to stainless steel welding wire can reduce the Cr content in welding fume but accelerate the formation rate of smoke.
Mortazavi S B et al. discovered that reducing the K content in welding materials and increasing the Li content can reduce the K2CrO4 content and subsequently reduce the Cr6+ content in welding fume through Li.
Additionally, Topham N et al. demonstrated that reducing the Na and K content in austenitic stainless steel welding materials and adding 30% tetraethyl silane (TEOS) in shielding gas can reduce the Cr (VI) content in stainless steel welding fume.
However, the de-alloying method of welding materials used to reduce harmful components in welding fume may not meet the requirements of mechanical properties, corrosion resistance, and wear resistance necessary for welding structures.
Currently, the degree of alloying in the base metal being used is very high. From low-carbon steel to low-alloy steel, and then to high entropy alloy, the level of alloying is increasing.
Simultaneously, adding alloy elements such as Mn, Cr, Ni, Mo, Co, and others to the welding materials (base material+welding wire) can significantly enhance the mechanical properties and corrosion resistance of the welding components, increase their service life, and expand the application range of metal materials.
As a result, it is often not practical to treat smoke and dust by dealloying welding materials in actual production.
Ventilation and smoke exhaust are currently the most effective methods of treatment in production, which mainly include two types of methods:
The first method involves installing local smoke extraction devices or using smoking welding guns on the welding station to control the further diffusion of welding smoke and harmful gases, and to control them from the source.
The second method involves improving the working environment of the welding workshop through comprehensive ventilation and displacement ventilation of the plant.
3.1 Local smoke extraction
Currently, the primary methods for local smoke extraction mainly include smoking welding guns and local ventilation and dust removal.
The principle of the smoking welding gun is illustrated in Figure 3. The smoking mouth generates suction to capture the smoke and dust, preventing their diffusion and environmental pollution.
Compared to other local processing equipment, smoking welding guns offer greater flexibility in terms of positioning and angle adjustment, enabling welders to operate with fewer restrictions.
Local ventilation involves using specialized dust hoods to directly extract welding smoke from the welding area and subsequently releasing the collected smoke outside after undergoing dust reduction treatment. The principle of local ventilation is depicted in Figure 4.
Research indicates that local ventilation is more efficient than general ventilation.
Flynn MR conducted a study comparing the dedusting effectiveness of a local ventilation system under three conditions: no indoor ventilation, natural wind, and mechanical ventilation. The results revealed that the fan combined with the local ventilation system had the highest dedusting efficiency.
In another experiment, Meeker JD evaluated a commercial local ventilation and dust removal equipment. The study found that the concentration of Mn in the air smoke decreased by 25%, particulate matter decreased by 40%, and Cr6+ decreased by 68% after using the equipment. Thus, local ventilation and dust removal is an effective ventilation method.
However, it should be noted that local smoke extraction equipment is only suitable for welding small-sized workpieces and has limited application in heavy structure welding workshops. This is due to the fact that the welding station for heavy structures is mobile, and the points of smoke and dust constantly change, making it challenging to consider the overall space using local dedusting.
3.2 General ventilation and displacement ventilation
General ventilation, also known as dilution ventilation, refers to the process of diluting indoor polluted air with clean air through the use of doors, windows, and roofs. This is done to reduce the concentration of harmful substances in indoor air and ensure that the indoor air environment meets air quality standards.
Its principle is shown in Fig. 5.
General ventilation is suitable for environments with low concentrations of harmful substances and is commonly used as an auxiliary mode for local ventilation and dust removal.
C. E. Feigley et al. studied and discussed the safety factor K in the formula for calculating the air volume for dilution ventilation, and proposed a more objective mixing factor Km based on experimental measurements.
Liu Siyan et al. conducted tests to evaluate the concentration of chemical hazards in a welding workshop before and after implementing mechanical ventilation treatment. Following ventilation treatment, the air content of manganese and its compounds, welding fume, ozone, carbon monoxide, and nitrogen oxides in the workshop decreased, with the most significant reduction found for manganese and its compounds at a concentration decrease of 82%.
Displacement ventilation is developed on the basis of general ventilation, and its principle is illustrated in Figure 6.
Due to the heat generated during the welding process, a stable temperature gradient is formed in the welding workshop, which reduces the wind speed and causes a temperature difference (ΔT=2~4 ℃) between the fresh air directly supplied to the indoor working area.
As a result, the colder air first descends under the influence of gravity and gradually spreads across the floor, forming a layer of fresh air. As the temperature increases, this fresh air rises, continuously taking away the polluted air.
Furthermore, fresh air is continuously supplied to the room through the air duct, while the air return opening above the workshop draws indoor air due to multiple factors.
The fresh air above the ground in the work area slowly moves upward, forming a uniform upward airflow. This gradually replaces the polluted air in the workshop, purifying the air.
The displacement ventilation dedusting method not only saves energy consumption but also provides higher purification efficiency. R. Nienel et al. conducted a study on the displacement ventilation system of large welding plants.
By analyzing the spatial distribution of particles generated during the welding process, they found that the concentration of particles in the personnel activity area at the lower part of the plant was significantly lower than the concentration at the upper part of the plant, thus demonstrating the effectiveness of displacement ventilation in discharging particles from the welding plant.
Currently, research on displacement ventilation mainly focuses on optimizing the air distribution, air supply parameters, and outlet position of displacement ventilation using CFD numerical simulation. This research aims to improve ventilation efficiency and provide theoretical guidance for optimizing the design of displacement ventilation.
(1) The generation and hazard of welding fumes are determined by complex physical and chemical processes, and comprehensive measures are required for their treatment.
(2) Complete control of welding fumes and other hazardous factors cannot be achieved through passive protection alone.
(3) The innovation of intelligent and automatic welding processes and welding systems has opened up a new path to achieving green, efficient welding and clean production.