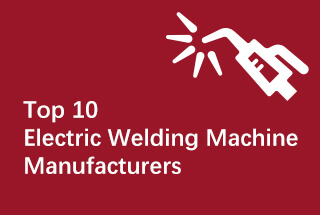
What makes welding magnesium alloys so challenging and exciting? In this article, you’ll explore innovative techniques like laser welding with silicon carbide, pulsed current plasma arc welding, and activated welding. Discover how these methods enhance joint strength, efficiency, and overall performance. Learn about groundbreaking approaches in fusion-adhesive welding and diffusion welding, and see how they revolutionize the field. This comprehensive guide will deepen your understanding of the latest advancements and practical applications in magnesium welding technology.
Over the past decade, due to significant impetus from energy conservation and environmental protection, the development of magnesium alloys and their welding technologies has accelerated more than ever before.
Breakthroughs constantly emerge from welding methods, materials, to equipment, providing unprecedented favorable conditions for the progression towards higher quality, more efficient, and cost-effective magnesium alloy welding production. This has greatly stimulated the industrialization of magnesium alloys.
Dissimilar metals such as magnesium and aluminum can be somewhat joined by methods like vacuum diffusion welding, explosive welding, and friction stir welding.
However, the bonding strength achieved is not ideal. The primary reason for this outcome is the formation of high-hardness, high-brittleness intermetallic compounds within the weld pool during the welding of the two materials.
Silicon carbide (SiC) particles are often combined with magnesium and aluminum alloys in the casting field to form composite materials. This combination refines the microstructure of the materials and enhances their mechanical properties comprehensively.
SiC is also frequently used in surface cladding processes. Based on the properties of SiC and its application in magnesium and aluminum composite materials, SiC is proposed as an intermediate layer for laser lap welding of magnesium and aluminum.
The aim is to use SiC particles as an intermediate layer for laser lap welding of magnesium and aluminum, thereby improving the performance of the magnesium-aluminum weld joint.
Comparing the direct laser welding of magnesium alloy and aluminum alloy with laser welding incorporating SiC particles, the macroscopic cross-section of the weld seam is illustrated in Figure 3.
Under the same laser power conditions, changes occur in the macroscopic morphology of the welding melt pool. Compared to direct laser welding, the addition of a SiC interlayer reduces the internal stirring capability of the melt pool and increases the melt width at the interface of magnesium and aluminum sheets.
Shear tests on the welded specimens reveal that the inclusion of SiC alters the microstructure of the melt pool, reduces the thickness of the intermetallic compound reaction layer, and increases the shear tensile resistance of the weld seam, which can be more than three times that of direct laser welding.
A. Direct Laser Welding
B. Laser Welding with a SiC Interlayer
Pulsed current plasma arc welding was used to seam weld a 5mm thick magnesium alloy sheet. The process schematic is shown in Figure 6.
The macroscopic photo of the weld seam is displayed in the figure. From the macro-structural image of the weld seam, the upper magnesium sheet exhibits the characteristic features of pulsed current plasma arc micro-hole welding – uniform back fusion, slight changes in the melt width dimensions on the top and bottom surfaces of the joint, which will be advantageous in reducing stress concentration and enhancing joint performance.
This method not only improves the welding quality of medium-thickness magnesium alloy sheets, but also simplifies the production process and enhances productivity, making it suitable for mass production.
Activated Tungsten Inert Gas (A-TIG) welding is a method that involves coating the parent material’s surface with an activator prior to welding. Under the same welding specifications, compared to conventional TIG welding, it can significantly increase the depth of the weld penetration.
When welding magnesium alloys, the depth of the AC TIG weld penetration noticeably increases after adding the developed activator, as shown in the figure.
Mechanical performance tests indicate that the strength of the A-TIG welded joint of magnesium alloys can reach 90% of the parent material, achieving a deep penetration and high-quality connection of magnesium alloys.
(a) Without activator
(b) With activator
The traditional active welding technique applies the activator on the surface of the welding test piece, which has a drawback of not being able to weld with filler wire. Therefore, a method of active filler wire welding has been proposed, in which the activator is applied to the surface of the welding wire, followed by filler wire welding, as shown in Figure 5.
The TIG filler wire welding of magnesium alloy active wire overcomes the difficulty of droplet transition during filler wire welding in traditional active welding techniques, enabling a smooth transition of droplets into the welding molten pool.
Concurrently, the activator enhances the welding penetration depth, making it an ideal high-efficiency welding method for magnesium alloys. Compared to the standard TIG filler wire welding method, this method can significantly improve the efficiency and penetration depth of magnesium alloy welding.
Figure 5 compares the welding penetration depths at currents of 60A and 90A. At 60A, the penetration depth increase rate (compared to the welding penetration depth of the active wire and the regular wire) can reach over 300%.
At a welding current of 90A, the penetration depth achieved with composite active wire welding is the greatest, with an increase rate of 243%. For a 5mm thick AZ31 magnesium alloy plate, it can be fully welded in a single pass.
Figure 5 Schematic diagram of welding
In response to the urgent demand for dissimilar metal material joining technology, a novel “fusion-adhesive” (or adhesive welding) joining technique is proposed.
This method organically combines continuous fusion welding technologies such as laser and plasma arc with adhesive technology, achieving an effective unity of mechanical, metallurgical, and chemical bonding.
Not only does it possess the advantages of traditional adhesive spot welding, but it also realizes the synergistic promotion of continuous fusion welding’s “line joining” and adhesive’s “surface joining. This significantly boosts the overall performance of the joint, providing a new direction for future connections of dissimilar materials.
Experiments were conducted on similar magnesium alloy plates using plasma arc adhesive welding technology. The process characteristics of plasma arc adhesive welding were explored, and the structure and performance of the weld seam were analyzed. This research provides significant guidance for further studying the process characteristics of plasma arc adhesive welding.
Plasma arc adhesive welding is a novel welding method that combines plasma arc welding and adhesive technology to join workpieces. The plasma arc has a high energy density and arc column temperature, providing concentrated heating to the workpiece and demonstrating a strong penetration ability.
This method can achieve a one-time full penetration on medium-thick plates, and at the same penetration depth, its welding speed is higher than TIG welding, thus improving welding productivity.
Furthermore, the plasma arc imparts less thermal input to the workpiece, and the weld seam cross-section is comparatively narrow with a large depth-to-width ratio, resembling a “wine glass” shape. The heat-affected zone is narrow, resulting in minor welding deformation.
Adhesive joints evenly distribute stress, avoiding stress concentration, and maintain good sealing. Adhesive bonding can eliminate the need for mechanical fasteners (like screws and nuts), and connection holes are not required, thereby preventing the reduction of the material’s effective cross-sectional area.
This method allows for the full utilization of the material’s strength, significantly reducing the overall structural weight. Additionally, the joint features insulation and corrosion resistance properties.
The image below depicts a schematic of the plasma arc adhesive welding process. The welded joint employs an overlapping joint method. The adhesive thickness is 0.1mm. Tests were conducted on magnesium alloy plasma arc adhesive welding under conditions without beveling or backside forced shaping protection.
As shown in Figure 19, the weld cross-section reveals that the molten pool is wider at the top and narrower at the bottom, resembling a “T” shape. The surface depression is minor, and the weld interior exhibits no evident defects such as porosity or cracks.
Mechanical property analysis indicates that the failure load of the plasma arc adhesive weld joint shows a significant enhancement compared to plasma arc welding and adhesive joints. Under the same loaded conditions, the plasma arc adhesive weld joint demonstrates a reduced tendency for thermal cracking.
The innovative laser adhesive welding technique has been applied to join dissimilar metals of magnesium and aluminum, achieving effective connections between thin sheets of these two metals.
This method significantly alters the distribution and characteristics of intermetallic compounds between magnesium and aluminum, thereby increasing the weld penetration depth and enhancing the strength of the welded joints.
During the laser adhesive welding process, the adhesive layer greatly impacts the entire weld pool. It not only changes the flow pattern of the entire molten pool but also directly affects the heat transfer during the welding process, thereby altering the conventional laser welding process.
The schematic diagram of the welding structure is shown in Figure 9, where an adhesive layer with a thickness of 0.1mm is brushed in the overlap area. The macroscopic morphology of the welded joint is shown in Figure 10.
Measuring the mechanical properties of the laser adhesive welded joint of dissimilar magnesium-aluminum metals reveals that its comprehensive mechanical performance reaches over 90% of the parent magnesium alloy, substantially meeting the current demand for the strength of magnesium-aluminum connections.
During the laser adhesive welding process, the addition of the adhesive layer accelerates the heat transfer between the magnesium alloy and the aluminum alloy, increasing the cooling speed of the magnesium alloy while indirectly preheating the aluminum alloy. This preheating to some extent enhances the aluminum alloy’s absorption rate for the laser.
Currently, the welding methods used for Mg/Al dissimilar metals are mainly fusion welding and solid phase welding, while diffusion welding with added intermediary transition metals is an effective method for dissimilar metal connections.
By adjusting the composition of the intermediary layer, control over the joint structure can be achieved, thereby improving joint performance. At present, there are few literature reports on Mg/Al diffusion welding connections with added intermediary transition metals.
The comparative analysis of Mg/Al joint under the conditions of inert gas protected diffusion welding, with and without transition metals, reveals the reason why the addition of intermediary transition metals enhances the shear strength of Mg/Al diffusion welded joint. The parent materials used in the experiment are 6061 aluminum alloy and AZ31B magnesium alloy.
Mechanical performance analysis indicates that the shear strength of Mg/Al direct diffusion welded joints can reach up to approximately 40MPa, while with the addition of suitable alloy transition metals, the shear strength of Mg/Al diffusion welded joints can achieve 100MPa.
The reason lies in the fact that direct diffusion welding of magnesium and aluminum inevitably results in the formation of numerous intermetallic compounds at the joint area.
However, the structure of Mg/Al diffusion welded joints with added alloy transition metals transitions from a network of eutectic forms into an intermediate phase composed of supersaturated magnesium-based solid solution and dispersed second-phase rich Al particles.
The addition of transition metals effectively prevents the direct contact of the magnesium-aluminum matrix, and the resultant dispersed particles significantly augment the joint strength.
With its high-quality coating, increased production efficiency, simplicity of operation, and energy-saving advantages, arc spraying technology has been a focal point of research since the invention of thermal spraying techniques.
Arc spraying involves using two wires, coated with the desired spray material, as consumable electrodes. The arc generated at the wire ends serves as a heat source to melt these wires.
Compressed air is then passed through the arc and the molten droplets, causing them to atomize and strike the substrate at a certain speed, thereby forming the coating.
By using arc spraying, weld joints can be protected comprehensively, making this method suitable for both homogeneous and heterogeneous metal weld joints. This paper discusses the process characteristics of arc spraying of magnesium alloys and its post-spraying treatment process, and introduces a newly developed magnesium-aluminum quasi-alloy coating. A pure aluminum coating is sprayed onto the surface of the magnesium alloy substrate to enhance its corrosion resistance.
The formation process of the coating inevitably results in a certain amount of porosity on both its surface and internally, which can become the origin of pitting. In order to further enhance the corrosion resistance of the coating, post-treatment sealing of the coating pores is an essential procedure.
Conductive pore sealing is adopted to meet the demands of specific industries. Metallic fillers are added to the organic sealing agent, epoxy resin, to form a blended conductive coating, completing the conductive pore-sealing process for pure aluminum coatings, while also investigating the corrosion resistance of organic conductive pore sealing.
The schematic diagram of conductive pore sealing is shown in Figure 11. Figure 12 illustrates the microscopic morphology of the copper powder-containing pore coating.
Coating resistance tests indicate that adding a certain amount of copper powder to the epoxy resin can make the pore-sealing coating conductive, and its conductivity is good. The corrosion resistance of the pore-sealing coating is studied using a room temperature immersion test.
Compared to the unsealed coating, the conductive pore-sealed coating shows good corrosion resistance.
The feasibility of utilizing heterogeneous metal wires for arc spraying is explored. Magnesium and aluminum wires are utilized as the two spraying wires, and intermetallic compound coatings are prepared on the surface of magnesium alloys to enhance their corrosion resistance, wear resistance, high-temperature strength, and oxidation resistance, thereby enabling their application in a broader range of fields.
High-purity aluminum wire with an aluminum content of more than 99.7% and high-purity magnesium wire with a magnesium content of more than 99.7% are used as the two poles of the spraying wire.
The surface morphology of the coating obtained by arc spraying is shown in Figure 13. The obtained coating is smooth and uniform, with small particles and no incompletely melted metal particles. The surface porosity is also minimal.
Microhardness measurements of the coating reveal an average microhardness of up to 200HV, while the average hardness of the arc-sprayed pure aluminum coating is only 67 HV.
The hardness of the compound produced by the reaction of magnesium and aluminum is higher than that of pure magnesium and aluminum, and the formation of a large number of intermetallic compounds results in an increase in the hardness of the coating.
After magnesium diffuses and reacts with aluminum, the residual oxides and MgAl2O4 phase particles dispersed in the matrix also contribute to the hardening effect.
The development and application of magnesium alloy, a novel structural material, has presented significant challenges to welding technology, while also broadening its potential uses. There remain many technical difficulties in the welding of magnesium alloys that await our exploration and resolution.
Overcoming these issues will lay a solid technical foundation for the practical application of magnesium alloys and the design and manufacture of magnesium alloy components.
The technological advancements discussed above will undoubtedly propel the welding technology level of magnesium alloy products, and promote the application of complex magnesium alloy components in industrial production.