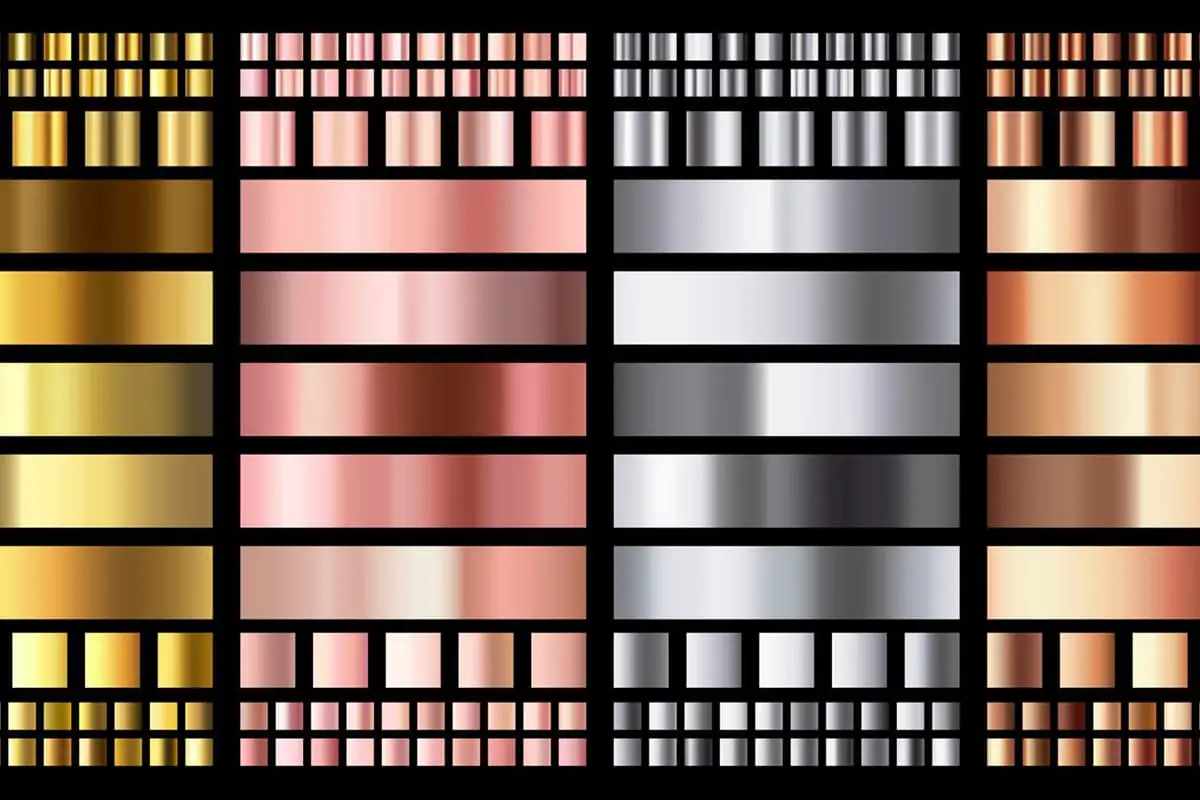
How does a metal maintain its strength and resist corrosion at high temperatures? Chromium-molybdenum steel, widely used in oil refining and chemical industries, achieves this through its unique alloy composition. This blog post explores the properties, heat resistance, and corrosion resistance of chromium-molybdenum steel. Discover the design, manufacturing considerations, and why this material is crucial for high-temperature, high-pressure applications. Dive in to learn how chromium-molybdenum steel can enhance your next engineering project.
Chromium-molybdenum steel, also known as medium temperature hydrogen-resistant steel, refers to the type of steel that is significantly enhanced in high-temperature strength and creep limit by adding alloy elements such as Cr (≤10%) and Mo.
It also has excellent hydrogen corrosion resistance and high-temperature performance, making it widely used in oil refining, chemical hydrogen devices, and high-temperature equipment.
It is one of the common types of steel used in pressure vessels.
This article discusses the characteristics of chromium-molybdenum steel materials and considerations in design, manufacturing, non-destructive testing, heat treatment, and start-up/shutdown operations in the context of the Jiutai Methanol Synthesis Project.
The addition of elements like chromium, molybdenum, and alum improves the steel’s resistance to high-temperature oxidation and high-temperature strength.
The mechanism of action is as follows: Chromium primarily exists in cementite (Fe3C), and the chromium dissolved in cementite enhances the decomposition temperature of carbides, preventing the occurrence of graphitization, thereby enhancing the heat resistance of the steel.
Molybdenum has a solid solution strengthening effect on ferrite and can also increase the stability of carbides, which benefits the high-temperature strength of steel.
The inclusion of an appropriate amount of vanadium allows the steel to maintain a fine-grained structure at higher temperatures, enhancing the steel’s thermal stability and strength.
Elements like chromium and molybdenum enhance the stability of carbides, preventing their decomposition, thereby reducing the chance of methane formation due to the reaction of carbides and precipitated carbon with hydrogen.
The addition of vanadium allows the steel to maintain a fine-grain structure at higher temperatures, significantly increasing the steel’s stability under high temperature and pressure conditions.
The temper embrittlement of chromium-molybdenum steel refers to the phenomenon where the steel’s impact toughness decreases when operated over a long period within the temperature range of 370°C to 595°C.
This is the exact temperature range within which our commonly used hydrogen equipment operates. Experimental studies have shown that in pressure vessel-grade chromium-molybdenum steel, temper embrittlement is most severe when chromium content is between 2% and 3%.
Elements such as phosphorus, antimony, tin, arsenic, silicon, and manganese have a significant impact on temper embrittlement. Embrittlement is reversible; materials that have severely embrittled can be de-embrittled through appropriate heat treatment.
Due to the addition of alloy elements such as chromium, molybdenum, and vanadium, the critical cooling speed of steel is reduced, enhancing the stability of supercooled austenite.
If the welding cooling speed is fast, the transformation from austenite to pearlite in the overheated zone of the heat-affected zone is unlikely to occur.
Instead, it transforms into martensite at lower temperatures, forming a quenched structure.
Under the combined action of complex residual stress at the welded joint and diffused hydrogen, the quenched structure in the weld area and heat-affected zone is highly susceptible to hydrogen-induced delayed cracking.
Under specific operational conditions, the selected materials should not only have superior hydrogen corrosion resistance but also effectively control the tendency toward temper brittleness.
They should also possess good weldability. Chemical composition determines the structure, structure determines performance, and performance determines use. Ultimately, the key lies in the control of chemical composition.
3.1.1 Measures Against Hydrogen Corrosion
Chromium molybdenum steel does not experience hydrogen corrosion even under high pressure at lower temperatures (~200°C). However, it may undergo hydrogen corrosion when operating in high-temperature, high-pressure hydrogen environments.
Typically, we select chromium molybdenum steel materials for specific operating conditions based on the Nelson curve, which corresponds to operating temperature and hydrogen partial pressure.
As can be seen from the Nelson curve, the higher the chromium and molybdenum content, the stronger the resistance to hydrogen corrosion.
In the curve, if the operating conditions of the vessel are above the solid line, it indicates the occurrence of hydrogen corrosion. If they are below the solid line, it indicates that hydrogen corrosion will not occur.
3.1.2 Measures to Control Temper Brittleness Tendency
By regulating the content of elements such as P, Sb, Sn, As, Si, Mn in the material, the temper brittleness tendency can be controlled.
The temper embrittlement sensitivity coefficient J of common steel and the temper embrittlement sensitivity coefficient x of the weld metal are usually used for this purpose. For commonly used 2.25Cr-1Mo, the following control indices are used:
In practical engineering applications, it is also necessary to control the content of residual elements Cu and Ni. The Cu content should not exceed 0.20%, and the Ni content should not exceed 0.30%.
3.1.3 Determination of Crack Sensitivity
Crack sensitivity is related to carbon equivalent, the value of which should be determined by the manufacturer based on the welding process evaluation.
The calculation method is: Ceq=C+Mn/6+(Cr+Mo+V)/5+(Ni+Cu)/15.
As the carbon equivalent value increases, the weldability of the steel deteriorates. When the Ceq value is greater than 0.5%, the sensitivity to cold cracking increases, and the welding and heat treatment processes will become stricter.
For commonly used Cr-Mo steel materials with 485Mpa ≤ UTS <550Mpa, Ceq is usually limited to approximately 0.48%.
When simulated welding and post-weld heat treatment are carried out on product weld test plates, the maximum carbon equivalent can be increased to 0.5%.
Due to the high hardening tendency of Cr-Mo steel, it is prone to delayed cracking and cracking at the corner welds.
Therefore, the structural design should pay attention to the following points:
3.2.1 Reduce the degree of restraint and reasonably design the joint structure.
3.2.2 The weld surface must not have an undercut.
3.2.3 Hole reinforcement should be implemented as a whole, and ring reinforcement structures should not be used.
3.2.4 Internal extension type nozzles should not be used.
3.2.5 The connection with accessories should adopt a double-sided full penetration structure, and corner welds should not be used.
3.2.6 The butt joint of the cylinder should preferably use a U-shaped groove.
Cr-Mo steel has a larger carbon equivalent value and generally has a tendency to cold crack to varying degrees. This can be prevented by the following measures:
3.3.1 Strictly control the hydrogen content in the welding rod and use a low-hydrogen basic electrode.
3.3.2 Preheating should be done before welding the equipment assembly. Through preheating, the cooling rate of the welding material can be reduced to prevent the formation of brittle hard structures.
The preheating temperature is determined by the welding process evaluation. Before the welding process evaluation, a welding crack test should be carried out on the sample to determine the preheating temperature, which should not be lower than the preheating temperature during the entire welding process.
At the same time, the interlayer temperature should be controlled not to be lower than the preheating temperature. Post-heating measures should be taken immediately after welding.
Every sheet of Cr-Mo steel used for the casing should undergo ultrasonic testing.
For high-temperature, high-pressure, thick-walled reaction vessels, after 100% radiographic inspection of the butt joints, ultrasonic testing and additional magnetic particle testing should be conducted on weld joints allowable for ultrasonic testing following heat treatment and hydrostatic testing.
Ultrasonic testing is more sensitive to cracks and defects than radiographic testing, hence it should be performed attentively, considering the timing for non-destructive testing.
During the manufacturing process of the vessel, hydrogen gas may infiltrate the metal, causing minor cracks in the steel, a phenomenon known as hydrogen embrittlement.
To prevent hydrogen embrittlement, post-weld dehydrogenation treatment should be promptly conducted.
Dehydrogenation treatment involves heating the weld and its adjacent parent material to a high temperature immediately after welding, thereby increasing the diffusion coefficient of hydrogen in the steel.
This encourages the outflow of oversaturated hydrogen atoms in the weld metal, thus inhibiting the occurrence of cold cracks. Dehydrogenation treatment may be deemed unnecessary if Post-Weld Heat Treatment (PWHT) is carried out immediately after welding.
Vessels of any thickness made from Cr-Mo should undergo overall post-weld heat treatment. Post-weld heat treatment of Cr-Mo steel not only eliminates residual stress but also enhances the mechanical properties of the steel, which is advantageous in resisting hydrogen corrosion.
Cr-Mo steel might succumb to brittle failure when its operating temperature is low or near the ductile-to-brittle transition temperature, and the stress reaches a certain level.
However, such failure is almost avoidable when the actual stress in the vessel is less than a fifth of the yield strength of Cr-Mo steel.
Therefore, for pressure vessels made from Cr-Mo steel, a procedure of increasing temperature before pressure during start-up and reducing pressure before temperature during shut-down should be adopted to prevent brittle failure.
When Implementing International Standard Cr-Mo Steel Materials
Due to the discrepancies in safety factor determination and calculation methods between domestic and international standards for permissible material stress, when using Cr-Mo steel materials from international standards, one should apply domestic rules for permissible stress calculation.
Taking SA387Cr.11G1.2 as an example, the calculation of its permissible stress is as follows:
First, obtain the tensile strength and yield strength at various temperatures for the material from ASME.
The permissible stress at room temperature is the smaller value between the room temperature tensile strength divided by 3.0 and the yield strength divided by 1.5.
Since there is no data on tensile strength at high temperatures domestically, the permissible stress at high temperatures is obtained by dividing the yield strength at high temperatures by 1.6.
If the calculated value is larger than the room temperature permissible stress, adopt the room-temperature value. Otherwise, use the calculated value.
The permissible stress of this material in ASME reveals that when the temperature exceeds 450℃, the permissible stress drops rapidly, at which point the creep limit governs the permissible stress.
Since ASME does not provide creep limit data above 450℃, and the safety factors for the creep limit in both domestic standards and ASME are consistent, we directly adopt the permissible stress from ASME. The specific permissible stress at the design temperature can be obtained using interpolation.
This article outlines some specific requirements for Cr-Mo steel materials. In detailed design work, it’s necessary to consider all aspects according to standard specifications, conduct comprehensive analysis, so as to achieve safe, economical, and rational design.