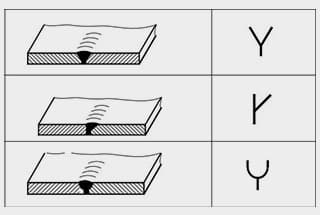
What determines whether two pieces of metal can be seamlessly joined? This article dives into the critical factors influencing the weldability of metal materials, from material composition to environmental conditions. Readers will gain insight into the principles of evaluating weldability, common testing methods, and how different metals respond under specific welding processes. Understanding these key points is essential for ensuring the integrity and performance of welded joints in various applications.
Metal weldability refers to the capability of homogeneous or heterogeneous materials to form a sound joint and meet the desired performance requirements during the manufacturing process. There are two types of weldability: process weldability and service weldability.
Process weldability is the ability of a metal or material to produce high-quality, dense, and defect-free welded joints that meet performance requirements under specific welding process conditions.
Weldability refers to the extent to which the welded joint and the overall welded structure meet various properties, including conventional mechanical properties.
There are four factors that can affect metal weldability: material factor, design factor, process factor, and service environment.
To evaluate weldability, the following principles should be considered: (1) Evaluate the likelihood of process defects in welded joints to provide a basis for designing a suitable welding process. (2) Assess whether the welded joint meets the structural performance requirements.
Experimental methods should meet the following principles: comparability, relevance, reproducibility, and economy.
A. Oblique V-Groove Welding Crack Test Method: This method is primarily used to evaluate the sensitivity of the carbon steel and low alloy high strength steel welding heat-affected zone to cold cracking.
B. Pin Test
C. Butt Welding Crack Test Method for Pressing Plate
D. Adjustable Restraint Crack Test Method
Understand the key steps in the experiment and analyze the factors that impact the stability of the results.
Answer:
The objective is to assess the vulnerability of the heat-affected zone in carbon steel and low alloy high strength steel welding to cold cracking.
In determining the sensitivity of the heat-affected zone in carbon steel and low alloy high strength steel welding to cold cracking, the factors that influence the stability of the results are the restraint of the welded joint, the preheating temperature, angular deformation, and incomplete penetration.
It is commonly accepted that if the surface crack rate in low alloy steel is less than 20%, it is considered safe for general welding structures.
Answer: influencing factors:
(1) Material Factors: This encompasses the base metal and the welding materials used, including welding rods for electrode arc welding, welding wires and fluxes for submerged arc welding, welding wires, and shielding gases for gas-shielded welding, among others.
(2) Design Factors: The design of the welded joint structures will impact the stress state, thereby affecting the weldability.
(3) Process Factors: Even for the same base metal, different welding methods and process parameters can have a significant impact on weldability.
(4) Service Environment: The service environment for a welded structure can vary, such as the working temperature, type of working medium, and load properties, among others.
Answer:
The use and welding properties of metal materials refer to the various properties specified by the technical requirements of the welded joint or overall welded structure, including conventional mechanical properties or properties under specific working conditions, such as low-temperature toughness, fracture toughness, high-temperature creep strength, long-term strength, fatigue performance, corrosion resistance, and wear resistance.
The weldability of a process refers to the ability of a metal or material to produce high-quality, dense, defect-free, and functional welded joints under specific welding process conditions.
For instance, low-carbon steel has good weldability, but its strength and hardness are not as high as those of high-carbon steel.
Answer:
(1) Cold cracks typically occur in the heat-affected zone;
(2) Evaluating the hardness of the joint is the most crucial factor in determining the likelihood of cold cracking, making it a useful indicator.
Typically, the welded joint includes the heat-affected zone.
The greater the difference between the hardness value of the welded joint and the base metal, the lower the toughness of the joint and its overall mechanical properties, making it more susceptible to brittle fracture and other hazards.
To minimize this difference and ensure the reliability of the welded joint, the welding process conditions must be carefully controlled.
While an increase in the carbon equivalent generally leads to an increase in the hardening of the heat-affected zone, this relationship is not always linear.
Low-carbon quenched and tempered steel is primarily utilized as a high-strength welded structural steel, with a low carbon content limit. The alloy composition is designed with weldability requirements in mind. The carbon content in low-carbon quenched and tempered steel is less than 0.18%, resulting in better welding performance compared to medium-carbon quenched and tempered steel.
The low-carbon martensite in the welding heat-affected zone of this steel results in a high martensite transformation temperature (MS) and self-tempering martensite, leading to a lower tendency for welding cold cracks compared to medium-carbon quenched and tempered steel. Good toughness can be achieved when fine low-carbon martensite (ML) or lower bainite (B) structures are obtained in the heat-affected zone.
The mixed structure of ML and low-temperature transformed bainite (B) provides the best toughness, with distinct crystal positions between bainite laths. The effective grain diameter is fine and has good toughness, and depends on the strip width. The mixing of ML and BL effectively divides the original austenite grains, promoting more nucleation positions for ML and limiting its growth. The effective grains in the ML + B mixed structure are the smallest.
Ni is an important element in the development of low-temperature steel, and its addition can improve the low-temperature properties of the steel. For example, 1.5Ni steel should have a reduced carbon content and strict limits on the contents of S, P, N, H, and O to prevent aging brittleness and tempering brittleness while increasing Ni. The heat treatment conditions for this kind of steel include normalizing, normalizing + tempering, and quenching + tempering.
In low-temperature steel, the strict control of carbon content and impurities such as S and P reduces the likelihood of liquefaction cracks. However, temper brittleness may still be a concern, and it is important to control the tempering temperature and cooling rate after welding.
Process characteristics of low-temperature steel welding:
The primary objective in welding low-temperature steel is to maintain the low-temperature toughness of both the weld and the heat-affected zone, in order to prevent cracks.
9Ni steel has a strong low-temperature toughness, but when welding with ferritic materials similar to 9Ni, the toughness of the weld is greatly diminished.
This can be attributed to the cast weld microstructure and the oxygen content in the weld.
However, 11Ni ferritic welding materials, which are similar to 9Ni steel, can achieve good low-temperature toughness through TIG welding. This is because TIG welding reduces the oxygen content in the weld metal to less than 0.05% of the base metal.
Hot cracks in welds of carbon quenched and tempered steel are often caused by the high carbon and alloy content, which results in a large liquid-solid interval and severe segregation. These factors increase the likelihood of hot cracks.
Cold cracks in medium carbon quenched and tempered steel are caused by the high carbon content and abundance of alloy elements, which result in a hardening tendency. Additionally, the low melting point of the steel results in martensite formation at low temperatures, which lacks the ability to self-temper and increases the likelihood of cold cracks.
Reheat cracks in the heat-affected zone can result in changes in performance.
Embrittlement in superheated zone
(1) Medium carbon quenched and tempered steel has a high carbon content, several alloy elements, and strong hardenability, making it susceptible to producing hard and brittle high carbon martensite in the welding superheated zone. The faster the cooling rate, the greater the formation of high carbon martensite and the more pronounced the tendency towards embrittlement.
(2) Despite high linear energy, it can be challenging to prevent the formation of high carbon martensite, which results in coarser and more brittle material.
(3) To improve the performance of the superheated zone, measures such as low linear energy, preheating, slow cooling, and post-heating are typically employed.
Heat-affected zone softening
When a quenching and tempering treatment is not possible after welding, it is necessary to take into account the softening of the heat-affected zone. The stronger the grade of quenched and tempered steel, the more severe the softening issue becomes. The extent and width of the softening zone are closely tied to the linear energy and method used in welding.
(1) In the hot cracks of the weld, the carbon and alloy element content of carbon quenched and tempered steel is high, leading to a large liquid-solid interval, severe segregation, and a high tendency for hot cracks.
(2) Cold cracking in medium carbon quenched and tempered steel is caused by its high carbon content and increased presence of alloy elements, resulting in an evident hardening tendency.
(3) The low melting point results in martensite formation at low temperatures that generally lacks the ability to self-temper, leading to a high tendency for cold cracks.
(4) Performance alterations in the heat-affected zone.
Embrittlement in superheated zone
(1) Medium carbon quenched and tempered steel is prone to producing hard and brittle high carbon martensite in the welding superheated zone due to its high carbon content, numerous alloy elements, and significant hardenability. The faster the cooling rate, the more high carbon martensite will be formed, and the more severe the tendency towards embrittlement will become.
(2) Despite having high linear energy, it is challenging to prevent the formation of high carbon martensite, which will make the material coarser and more brittle.
(3) To improve the performance of the superheated zone, measures such as low linear energy, preheating, slow cooling, and post-heating are usually employed.
Heat-affected zone softening
When welding is completed and quenching and tempering treatment cannot be performed, it is necessary to take into account the softening of the heat-affected zone (HAZ).
The more the strength grade of the quenched and tempered steel is increased, the more pronounced the softening issue becomes.
The extent and width of softening are closely linked to the energy of the welding line and the welding method used.
The welding method that utilizes a more focused heat source is more advantageous in reducing softening.
(1) Medium carbon quenched and tempered steel is typically welded in its annealed state. Upon completion of the welding process, uniform welded joints with desirable properties can be achieved through an overall quenching and tempering treatment.
(2) When welding is performed after quenching and tempering, it is often challenging to address the degradation of the heat-affected zone’s performance.
(3) The pre-welding state determines the nature of the problems and the necessary steps to be taken in the process.
The weldability characteristics of Q345 steel are analyzed, and the corresponding welding materials and welding process requirements are given.
Answer: Q345 steel is a type of hot-rolled steel with a carbon content of less than 0.4% and excellent weldability.
Generally, preheating and precise control of the welding heat input is not necessary. However, it is important to consider the potential effects on the material.
Regarding the brittle and hard properties, when Q345 steel is continuously cooled, the pearlite transformation shifts to the right, resulting in ferrite precipitation under rapid cooling, leaving carbon-rich austenite to transform into pearlite too late. This transformation into bainite and martensite with high carbon content leads to a hardening effect. However, due to its low carbon content and high manganese content, Q345 steel has good resistance to hot cracking.
By adding V and Nb to Q345 steel, the stress crack in the welded joint can be eliminated through precipitation strengthening.
It is important to note that coarse grain embrittlement may occur in the superheated zone of the heat-affected zone when heated above 1200 ℃, resulting in a significant reduction in toughness. However, annealing Q345 steel at 600 ℃ for 1 hour greatly improves its toughness and reduces the tendency for thermal strain embrittlement.
For welding material selection, the following options are recommended:
It is recommended to preheat the material to a temperature of 100 to 150 ℃. For post-weld heat treatment, arc welding does not typically require it, or it can be tempered at 600 to 650 ℃. Electroslag welding, on the other hand, requires normalizing at 900 to 930 ℃ and tempering at 600 to 650 ℃.
What is the difference in weldability between Q345 and Q390? Is the welding process of Q345 applicable to the welding of Q390 and why?
Answer: Q345 and Q390 are both hot-rolled steels that have a similar chemical composition.
The only difference between Q345 and Q390 lies in the Mn content, with Q390 having a higher concentration. As a result, Q390 has a higher carbon equivalent compared to Q345.
This results in an increased hardenability and a greater likelihood of cold cracks in Q390 when compared to Q345. However, their weldability remains similar.
It should be noted that the welding process used for Q345 may not be suitable for Q390 due to its higher carbon equivalent and wider heat input, which could result in overheating and severe embrittlement in the joint area if the heat input is too high, or cold cracks and brittle behavior if the heat input is too low.
What is the principle of selecting welding materials when welding low-alloy high strength steel? What is the effect of post-weld heat treatment on welding materials?
Answer: The principle for selection should take into account the impact of the microstructure of the weld and the heat-affected zone on the strength and toughness of the welded joint.
Since post-weld heat treatment is usually not performed, it’s crucial that the weld metal have similar mechanical properties to the base metal in its as-welded state.
For medium carbon quenched and tempered steel, the choice of welding materials should be based on the stress conditions of the weld, its performance requirements, and any planned post-weld heat treatment.
For components that will undergo treatment after welding, the chemical composition of the weld metal should be comparable to that of the base metal.
Analyze the possible problems during the welding of low-carbon quenched and tempered steel.
This post provides a brief overview of the key aspects of welding low-carbon quenched and tempered steel.
What is the recommended range for controlling the welding heat input of typical low-carbon quenched and tempered steel such as 14MnMoNiB, HQ70, and HQ80?
When preheating is necessary, why are there minimum temperature requirements and how can the maximum preheating temperature be determined?
Answer: Embrittlement can easily occur during the welding process. The thermal cycle during welding can reduce the strength and toughness of the heat-affected zone.
Welding process features: Typically, post-weld heat treatment is not necessary. A multi-layer process is used and a narrow weld bead is employed rather than the transverse swing strip transportation technique.
The welding heat input for typical low-carbon quenched and tempered steel should be controlled to be less than 0.18% WC, and the cooling rate should not be accelerated. When the WC is greater than 0.18%, the cooling rate can be increased to reduce the heat input.
The welding heat input should be kept below 481 kJ/cm. If the maximum allowable welding heat input is reached and cracks cannot be avoided, preheating measures must be taken.
If the preheating temperature is too high, it will not prevent cold cracks from occurring. On the other hand, if the cooling rate between 800 to 500°C is slower than the critical cooling rate of brittle mixed structures, the toughness of the heat-affected zone will decrease.
Therefore, it is important to avoid unnecessary increases in preheating temperature, even at room temperature. As a result, there is a minimum preheating temperature.
The maximum allowable welding heat input of steel should be determined through experiments and then, based on the cold crack tendency at the maximum heat input, it should be decided if preheating and preheating temperature, including the maximum preheating temperature, are necessary.
What is the difference in the welding process between quenched and tempered and annealed medium carbon quenched and tempered steel of the same brand? Why is medium carbon quenched and tempered steels generally not welded in the annealed state?
When welding in the quenched and tempered state, it is crucial to follow proper procedures to prevent delayed cracks and eliminate the hardened structure in the heat-affected zone. This includes preheating, maintaining control of interpass temperatures, conducting intermediate heat treatment, and timely tempering after welding.
To minimize softening of the heat effect, it is recommended to adopt a method with high energy density and heat concentration, and to use as small a welding heat input as possible.
For welding in the annealed state, common welding methods can be employed.
When selecting materials, it is important to ensure consistency in the quenching and tempering treatment specifications of the weld metal and base metal, as well as consistency in their main alloy.
In the case of quenching and tempering, a high preheating temperature and interlayer temperature can help to avoid cracking before the treatment.
Due to the high hardenability and hardenability of medium carbon quenched and tempered steel, improper welding in the annealing state may result in delayed cracks.
A complex welding process is typically required, and auxiliary processes such as preheating, post-heating, tempering, and post-weld heat treatment can help ensure the joint’s performance and longevity.
Is there any difference in the welding process and material selection when low-temperature steel is used at – 40 ℃ and normal temperature? Why?
Answer: In order to avoid low-temperature embrittlement and thermal cracking in welded joints made of low-temperature steel, it is important to minimize the presence of impurity elements in the materials.
To control the weld composition and structure, it is important to select appropriate welding materials that will form fine acicular ferrite and a small amount of alloy carbide, thus ensuring certain AK requirements at low temperatures.
When using SMAW (Shielded Metal Arc Welding) in low temperature welding, the use of small linear energy welding can prevent overheating of the heat affected zone and reduce the formation of coarse M and WF (Weld Fracture). To further reduce weld bead overheating, fast multi-pass welding can be applied.
For the SAW (Submerged Arc Welding) process, the use of vibration arc welding method can prevent the formation of columnar crystals.
What are the differences in strengthening methods and main strengthening elements between hot rolled steel and normalized steel, and what are the differences in weldability between them? What problems should be paid attention to when formulating the welding process?
Answer: the strengthening methods of hot-rolled steel are:
(1) Solid Solution Strengthening: The main strengthening elements in this process are Mn and Si.
(2) Fine Grain Strengthening: The primary strengthening elements in this process are Nb and V.
(3) Precipitation Strengthening: The main strengthening elements in this process are Nb and V.
Strengthening mode of normalized steel:
Weldability: Hot rolled steel contains a limited number of alloy elements and has a low carbon equivalent, which reduces the likelihood of cold cracking.
Normalized steel contains a higher amount of alloy elements, which increases its hardenability and reduces the likelihood of cold cracking. It also has a low carbon equivalent.
However, heating hot rolled steel above 1200 ℃ can lead to the formation of coarse grain embrittlement, which significantly decreases its toughness.
On the other hand, under the same conditions, the V precipitate in the coarse grain region of normalized steel is primarily in a solid solution state, leading to a weakening of its ability to inhibit growth and refine the microstructure. This can result in the appearance of coarse grains, upper bainite, and M-A, leading to a decrease in toughness and an increase in aging sensitivity.
When planning the welding process, the choice of welding method should be made based on factors such as the material structure, plate thickness, required service performance, and production conditions.
Low carbon quenched and tempered steel and medium carbon quenched and tempered steel belongs to quenched and tempered steel. Are their embrittlement mechanisms in the welding heat-affected zone the same?
Why does welding low carbon steel in its quenched and tempered state ensure good welding quality, while medium carbon steel in the same state often requires post-weld heat treatment?
Answer: Low carbon quenched and tempered steel: When subjected to repeated cycles of increasing T8/5, low carbon quenched and tempered steel becomes brittle due to the coarsening of austenite and the formation of upper bainite and M-A constituents.
Medium carbon quenched and tempered steel: This type of steel has a high carbon content and several alloying elements, which results in a strong hardening tendency, low martensitic transformation temperature, and no self-tempering process.
As a result, welding in the heat-affected zone can cause a significant amount of M structure formation and potential brittleness.
In contrast, low carbon quenched and tempered steel typically benefits from moderate to low heat input during welding, while the best results for medium carbon steel are achieved through the use of high heat input during welding and prompt post-weld heat treatment.
What is the difference between the weldability characteristics of Pearlite Heat-resistant Steel and low-carbon quenched and tempered steel?
What is the difference between the principle of selecting welding materials for Pearlite Heat-resistant Steel and strength steel? why?
Answer: Cold cracks can occur in both pearlite heat-resistant steel and low-carbon quenched and tempered steel.
The heat-affected zone and reheat cracks can undergo hardening and embrittlement during heat treatment or prolonged use at high temperatures.
However, in low-carbon quenched and tempered steel, hot cracks may occur in high nickel and low manganese steel. Additionally, improper selection of materials can lead to hot cracks in Pearlitic Heat-resistant Steel.
When selecting Pearlitic heat-resistant steel, it is important to not only consider the material’s strength but also the principles for using the joint at high temperatures.
It is also crucial to ensure that the welding materials are dry, as Pearlitic Heat-resistant Steel is used at high temperatures and must meet certain strength requirements.
Welding of Stainless Steel and Heat-Resistant Steel
Some concepts:
Chromium Equivalent: The relationship between the composition and structure of stainless steel is depicted in a diagram. The elements that form ferrite are transformed into a sum of chromium (Cr) elements, taking into account their level of influence. This sum is referred to as the Chromium Equivalent, with a coefficient of 1 for chromium.
Nickel Equivalent: In the same diagram, the elements that form austenite are transformed into a sum of nickel (Ni) elements, considering their level of influence. This sum is referred to as the Nickel Equivalent, with a coefficient of 1 for nickel.
4750°C Embrittlement: This form of embrittlement occurs when high-chromium ferritic stainless steel is heated for a prolonged period at temperatures between 400°C and 540°C. It is called 4750°C brittleness because its most sensitive temperature is around 475°C. At this temperature, the strength and hardness of the steel increase, while its plasticity and toughness decrease significantly.
Solidification Mode: The solidification process starts with crystallization, followed by the completion of the process with either the γ or δ phase.
Stress Corrosion Cracking: This refers to cracks that form in a weak corrosive medium below the material’s yield point, under the combined action of stress and the corrosive medium.
σ Phase Embrittlement: The σ phase is a brittle, hard, and non-magnetic intermetallic compound phase with a complex and compositional crystal structure.
Intergranular Corrosion: This refers to selective corrosion near the grain boundaries.
Chromium Deficiency Mechanism: The supersaturated solid solution of carbon diffuses to the grain boundaries, forming chromium carbide (Cr23C16 or (Fe, Cr)C6) with chromium near the boundary and precipitating at the grain boundary. Since carbon diffuses much faster than chromium, it is too late for chromium to supplement from within the crystal to near the grain boundary, resulting in a mass fraction of Cr in the layer adjacent to the grain boundary that is less than 12%, which is referred to as “chromium deficiency.”