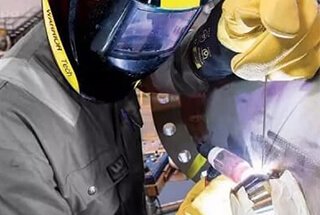
Choosing the right welding materials is critical for ensuring strong, durable welds. This guide explores the principles and best practices for selecting welding materials based on the performance requirements of welded joints, manufacturing process considerations, and economic factors. From carbon steel to stainless steel, the article provides detailed criteria for various materials, helping you make informed decisions to achieve optimal welding results. Whether you’re dealing with high-temperature conditions or looking for cost-effective solutions, you’ll find valuable insights to enhance your welding projects.
To obtain high-quality welded joints, the selection of welding materials should be reasonable. Due to the great differences in the operating conditions of welded components, the material properties and composition of the base material vary greatly, and the manufacturing process of the components is complex and diverse.
Therefore, it is necessary to comprehensively consider various aspects to determine the corresponding welding materials.
The selection of welding materials should follow the following principles:
(1) Meet the requirements of welding joint performance, including room temperature and high temperature short-term strength, bending performance, impact toughness, hardness, chemical composition, and special performance requirements for joints in technical standards and design drawings, such as long-term strength, creep limit, high-temperature oxidation resistance, corrosion resistance, etc.
(2) Meet the requirements of manufacturing process performance and welding process performance of welded joints.
The components that make up the welded joint inevitably need various forming and cutting processes during the manufacturing process, such as stamping, rolling, bending, turning, planing, etc., requiring the welded joint to have certain plastic deformation ability, cutting performance, high-temperature comprehensive performance, etc.
The welding process requires good process performance of the welding material and the ability to resist defects such as cracking according to the differences in welding properties of the base material.
(3) Reasonable economy.
While meeting the minimum requirements for various performance and manufacturing performance mentioned above, inexpensive welding materials should be chosen to reduce manufacturing costs and increase economic benefits.
For example, when welding low-carbon steel for important components using manual arc welding, alkaline coated electrodes should be preferred because they are fully deoxidized, desulfurized, and have low hydrogen content, with good crack resistance and impact toughness of the weld metal.
For some non-critical components, acidic electrodes can be used because they can still meet the performance requirements of non-critical components, have good processability, and are cheap, which can reduce manufacturing costs.
When selecting welding materials for carbon steel and low alloy steel (including low alloy heat-resistant steel and low alloy high-strength steel), the following factors should be considered:
(1) Principles of equal strength and equal toughness
For pressure-bearing components, strength calculations are usually based on the allowable tensile stress of the material.
The allowable tensile stress is related to the lower limit of the standard tensile strength of the material, that is, the allowable stress [σ] = σb / nb (the values of nb vary according to different standards), where [σ] is the allowable tensile stress of the material, σb is the lower limit of the standard tensile strength of the material, and nb is the safety factor (the values of nb vary according to different standards).
Therefore, as a part of the component, the tensile strength of the weld should not be less than the lower limit of the standard tensile strength of the base material.
At the same time, attention should be paid to the fact that the tensile strength of the deposited metal of the welding material should not be much higher than the tensile strength of the base material, which may lead to a reduction in the plasticity of the weld and an increase in hardness, which is not conducive to subsequent manufacturing processes.
Although strength calculations only consider the tensile strength of the material and various process evaluation standards do not require the yield strength of the weld, when selecting welding materials, the yield strength of the deposited metal of the welding material should also be considered not to be lower than the yield strength of the base material, and attention should be paid to ensuring a certain yield-to-tensile strength ratio.
When the joint operates at high temperatures, the allowable stress calculation is usually based on the lower limit of the short-term high-temperature tensile strength specified by the material at the working temperature (or design temperature), that is, [σt] = σbt / nb, where [σt] is the allowable stress calculated based on the lower limit of the short-term high-temperature tensile strength at temperature t, σbt is the lower limit of the short-term high-temperature tensile strength specified by the material at temperature t, or the allowable stress is calculated based on the long-term strength and creep limit of the material at the working temperature, that is, [σD t] = σDt / nD, where [σDt] is the allowable stress calculated based on the long-term strength at temperature t, σDt is the long-term strength of the material at temperature t, and nD is the safety factor (the values of nD vary according to different standards).
Therefore, when selecting welding materials for high-temperature operating welded joints, their short-term high-temperature tensile strength or long-term strength should not be lower than the corresponding values of the base material.
For carbon steel and ordinary low alloy steel, the selection of welding materials mainly considers the tensile strength of the welding material, and the chemical composition match between the deposited metal and the base metal may not be considered.
However, for Cr-Mo heat-resistant steel, the selection of welding materials should not only consider its equal strength but also consider matching the alloy elements to ensure that the comprehensive performance of the welded joint is consistent with the base metal.
In special cases where components are designed based on the yield strength of the material, the principle of equal yield strength should be an important consideration factor.
Due to different operating conditions of components, brittle fracture often occurs during operation due to insufficient toughness, especially for components working at low temperatures or high-strength thick-walled components.
Therefore, relevant standards have clear requirements for the impact toughness of welded joints. When selecting welding materials, it is necessary to ensure that the impact toughness of the weld meets the requirements of the relevant standards.
However, different standards have different requirements for the impact toughness of the joint. The Steam Boiler Safety Supervision Regulations stipulate that the impact toughness of the welded joint shall not be lower than the lower limit of the impact toughness specified by the base material.
If the base material does not have an impact toughness index, it shall not be lower than 27J. The Steel Pressure Vessel Standard GB150 specifies that the impact toughness value of the joint is determined according to the lowest tensile strength of the steel. For carbon steel and low alloy steel, the minimum impact toughness of the joint is:
For low-temperature vessels, the impact toughness value should not be lower than the lower limit of the specified value of the base material.
However, ASME regulation VIII-1 determines whether the joint needs to ensure impact toughness performance based on the strength level, thickness, working temperature, and the ratio of design stress to allowable stress of the material.
If the joint has impact toughness requirements, the minimum guaranteed value of impact toughness is specified based on the strength level and thickness of the material.
In summary, when selecting welding materials, we should determine the requirements for the impact toughness of the joint according to the design, manufacturing, and inspection standards of the product, and select suitable welding materials to meet the standard requirements, that is, the requirements for use performance.
When considering impact toughness requirements, attention should be paid to the design temperature and operating temperature of the structure.
If the operating temperature is equal to or higher than room temperature, only the room temperature impact toughness of the joint needs to be maintained; if it is below room temperature, the impact toughness value specified in the standard or drawing at the corresponding temperature should be ensured.
Of course, the performance of the welded joint is not only related to the welding materials but also related to the specific welding process.
Therefore, the selection of welding materials for the joint is a complicated issue.
(2) Consider the requirements and impacts of manufacturing processes
After welding components, they often need to undergo various forming processes such as rolling, pressing, bending, and calibration.
Therefore, welded joints and base materials should have a certain deformation capacity, especially cold deformation capacity, which is measured by the bending test of the joint. Many standards have made clear requirements for the bend test of welded joints of various materials.
The “Steam Boiler Safety Technical Supervision Regulations” stipulate that the bending shaft diameter D=3a (a is the thickness of the specimen) during the bend test, and the carbon steel is qualified for a bending angle of 180°, while the low-alloy steel is qualified for 100°.
GB150-99 Steel Pressure Vessels and ASME Section IX stipulate that when any material is subjected to the bend test, the bending shaft diameter D=4a, and the bending angle of 180° is qualified.
Therefore, when selecting welding materials, the bending performance of the weld metal should meet the requirements of the above standards.
In addition, the selection of welding materials should also consider the effects of post-weld heat treatment processes (such as post-weld annealing, normalizing, quenching and tempering, etc.) on the weld metal properties.
It should be noted that post-weld annealing heat treatment, especially post-weld normalizing, can cause significant changes in the weld metal properties. When the welding component is relatively thin, stress relief heat treatment after welding is not necessary.
As long as the performance of the weld metal in the as-welded condition meets the relevant requirements. For thick-walled welding components, according to relevant manufacturing standards, stress relief annealing should be carried out after welding if the wall thickness exceeds a certain limit.
Different heating temperatures and holding times during heat treatment will lead to different changes in the properties of the weld metal.
In engineering, the Larson-Miller parameter, also known as tempering parameter, is used to discuss the joint properties affected by the heating temperature and holding time of stress relief annealing. The formula for the tempering parameter is:
[P]=T(20+logt)×10-3
Where T is the absolute temperature in Kelvin, and t is the time in hours.
Tempering parameters〔P〕=T(20+Logt)×10-3
Generally, as the [P] value increases, the tensile strength and yield strength of the weld metal decrease, elongation increases, and impact toughness fluctuates.
Figures 1 and 2 show the relationship between the tempering parameters of deposited metal and mechanical properties for welding rods CMA96 and CMA106, respectively.
Therefore, when selecting post-weld heat treatment for welding materials, it is necessary to consider whether the mechanical properties of the deposited metal at the corresponding [P] value meet the relevant standards.
It should be noted that when the welded joint needs to undergo hot stamping, hot calibration, hot rolling, or other hot forming processes after welding, if the heating temperature reaches above the AC3 temperature of the material and is kept for a period of time before cooling in still air, the cooling rate during normalizing process is much slower than that during welding process.
The normalized process will result in the weld metal staying longer at 800-500℃ than during the welding process.
Allowing steel to be heated above AC3 during normalizing process will cause complete austenitization, followed by recrystallization during cooling, which destroys the originally overcooled structure of the weld metal and greatly reduces the weld strength.
The most severe reduction can exceed 100 MPa. Therefore, for welded joints that need to undergo hot forming processes, the selected welding material should have a strength level 50-100 MPa higher than that of the welded material in the as-welded condition or with stress relief treatment.
For example, for 19Mn6, the submerged arc welding wire in the as-welded condition is H08MnMO, while for normalized and tempered conditions, H08Mn2Mo should be used instead.
For SA675, a 300,000 kW steam drum lifting rod material with a minimum tensile strength of 485 MPa, J507 welding rod is normally used for manual arc welding.
However, in the case of welded joints on bend sections that undergo hot bending and normalizing treatment, J607 is recommended based on experimental results.
When selecting welding materials for welded joints subjected to normalizing and tempering treatments, not only should the strength be considered to increase by 50-100MPa above the usual conditions but also the chemical composition of the weld metal should be equivalent to that of the base material. This is because the composition and content of the alloy determine the AC3 temperature of the material.
If the chemical composition of the weld metal and the base material differ greatly, the AC3 temperature will also differ greatly. When the base material and the weld metal are normalized together, it is impossible to determine the appropriate normalization temperature.
In addition, if the welded joint needs quenching and tempering treatment, the impact of such treatment on the performance of the joint should also be considered. The strength of the welding material for quenched and tempered joints may be lower than that for normalized and tempered joints.
For example, for BHW35, H10Mn2NiMo is used after electric arc welding and normalizing, while for quenching and tempering treatment, H10Mn2Mo can be used instead.
Consider the weldability of materials and the metallurgical characteristics of welding methods. Different materials have different weldability, and there are different requirements for certain key element content. When selecting welding materials, the weldability of the material should be considered.
For example, the weld metal of 2.25Cr-1Mo heat-resistant steel may experience so-called temper embrittlement phenomenon when holding or slowly cooling in the temperature range of 332-432℃, which causes a significant increase in the brittle transition temperature of the weld metal.
Studies have shown that the sensitivity of temper embrittlement of this type of weld metal is caused by P, As, Sb, and Sn impurities deflecting at grain boundaries. It is generally believed that the low-temperature temper embrittlement of the weld metal is related to the P and Si content. The P and Si contents in the weld metal must be reduced to P≤0.015% and Si ≤0.15%.
Therefore, for Cr-Mo heat-resistant steel submerged arc welding, HJ350 welding flux with medium manganese and medium silicon should be selected instead of HJ431 matched with H08Cr3MnMoA wire. The sensitivity of temper embrittlement of the weld metal depends on the alloy series of the weld metal. Similarly, C-Mo, Mn-Mo, and Mn-Ni-Mo series weld metals also have temper embrittlement issues.
Welding materials with matching HJ350 welding flux should be used for submerged arc welding wire of the aforementioned series to reduce the Si content in the weld metal. For example, the submerged arc welding wire H08Mn2Mo should be matched with HJ350 welding flux for welding BHW35. If higher impact toughness of the weld metal is required, the welding flux should also be HJ250 or HJ250+HJ350 mixed flux.
However, for low-silicon welding wires such as H08MnA and H10Mn2, there is no temper embrittlement phenomenon in the weld metal. These two types of welding wires should be used with high silicon and high manganese welding flux HJ431 when welding 20# or 16Mn steel.
By using high-manganese and high-silicon welding flux, the welding pool will be siliconized, and a certain amount of silicon content in the weld metal is beneficial to the deoxidation process of the weld metal, preventing the occurrence of pores. When selecting welding materials, the metallurgical characteristics of different welding methods should also be considered.
For example, for gas metal arc welding with CO2 or CO2+Ar as shielding gas, there is no metallurgical reaction between the flux or welding wire and the metal during the welding process. However, there may be a reaction between CO2 and the metal elements to form iron oxide FeO.
Therefore, the welding wire must contain appropriate amounts of silicon and manganese to reduce the reduction reaction and ensure the formation of dense weld structure. In tungsten inert gas welding, there is no oxidation-reduction reaction, and the filler wire and base material are actually remelted.
Therefore, the argon arc welding wire must be fully deoxidized, and boiling steel materials should not be used. Otherwise, pores will occur in the weld. Calm steel material should be used instead, and it is unnecessary to have a certain Si and Mn content in the welding wire
For example, when using 15CrMo heat-resistant steel for welding with argon arc welding, H08CrMo welding wire should be selected; whereas for fusion electrode gas shielded welding, H08CrMnSiMo welding wire should be chosen.
The principle of the same strength of welding materials and parent materials is not entirely applicable to austenitic stainless steel. When used in corrosive environments with no specific strength requirements, the main concern is the anti-corrosion properties of the welded joint.
If used in high-temperature and high-pressure conditions with short-term work, certain high-temperature and short-term strength is required, while long-term work requires sufficient durable strength and creep limit of the weld metal.
For example, when SA213-TP304H pipes are used in high-pressure and high-temperature conditions, E308H welding materials should be selected.
When welding austenitic stainless steel, the selection of welding materials mainly considers that the chemical composition of the deposited metal should be equivalent to that of the base material.
As long as the chemical composition of the deposited metal of the welding material is the same as that of the base material, the performance of the weld metal can be equivalent to that of the base material, including mechanical properties, corrosion resistance, etc.
Special attention should be paid to the special requirements for corrosion resistance under the manufacturing process conditions or drawings.
In order to prevent intergranular cracking during welding, it is best to use stainless steel welding materials with low carbon (ultra-low carbon) content and containing Ti and Nb.
If the SO2 content in the coating or flux of the welding rod is too high, it is not suitable for welding austenitic steels with high nickel content.
To prevent hot cracks in the weld (solidification cracks), the content of impurities such as P, S, Sb, and Sn should be controlled, and it is preferable to avoid the formation of a single-phase austenite structure in the weld metal as much as possible.
Although many materials suggest that the ferrite content in the austenitic stainless steel weld metal is beneficial to reduce the tendency of weld metal cracking, a large amount of pure austenitic stainless steel weld metal has been used for many years and the joints have performed well.
Proper ferrite content is advantageous for corrosion resistance in certain media, but harmful to the impact of weld metal under low-temperature conditions.
Taking comprehensive factors into consideration, it is generally desirable that the ferrite content in austenitic stainless steel should be between 4% and 12%, because a ferrite content of 5% can achieve satisfactory resistance to intergranular corrosion.
The ferrite content in the weld can be estimated using the chemical composition of the weld metal, converted into Cr equivalent and Ni equivalent, through a microstructure chart.
Commonly used charts include WRC-1988, Esptein and DeLong.
The WRC-1988 chart is suitable for 300 series stainless steel and duplex stainless steel, but not applicable to materials with N>0.2% and Mn>10%. The Epstein chart is suitable for 200 series nitrogen-strengthened austenitic stainless steel with Mn<1.5% and N<0.25%.
When selecting austenitic stainless steel welding materials, attention should be paid to the influence of welding methods on the chemical composition of the deposited metal. Tungsten inert gas welding has the least effect on the change of chemical composition of the weld metal, and the other changes except for C and N are small in the non-diluted weld metal.
In particular, C loss is the largest. For example, when the C content of the electrode is 0.06%, the content in the non-diluted deposited metal of argon arc welding is 0.04%, and the N content in the weld metal increases by about 0.02%.
The Mn, Si, Cr, Ni, and Mo content in the deposited metal may undergo slight changes during melting electrode gas shielded arc welding, while the loss of C is only 1/4 of that of argon arc welding, and the increase in N content is much higher. The amount of increase differs according to different welding processes, up to a maximum of 0.15%.
During manual arc welding and submerged arc automatic welding, the alloy elements in the weld metal are jointly affected by the coating, flux, welding wire, and electrode.
Especially for welding materials with alloy element transition through the coating or flux, it is impossible to estimate the chemical composition of the weld metal by the chemical composition of the welding wire or electrode.
Of course, the ferrite content in the weld can be estimated from the alloy content in the weld metal, but this estimation value has a certain deviation from the actual value because the cooling rate during the welding process also affects the ferrite content.
It is generally agreed that if the alloy element content in the weld metal is exactly the same, the ferrite content will differ depending on the welding method.
The ferrite content is highest in strip cladding and lowest in argon arc welding. Even with the same strip cladding, it was found that the ferrite content at the start and end of the weld was about 2-3% lower than that of the middle segment.
With the standardization of stainless steel materials and welding materials, the selection of austenitic stainless steel welding materials has become simple. Corresponding welding material grades can be selected based on the stainless steel material grades, such as selecting E316 electrodes for SA240-316 stainless steel.
For martensitic stainless steel, it is best to use welding materials that are the same as the base material. For example, 1Cr13 steel should use E410 series welding materials, and the welding electrode number for manual arc welding is G217.
However, the weld metal structure of ordinary welding materials corresponding to 1Cr13 has coarse martensite and ferrite, which is hard and brittle and prone to cracking. In addition, the weldment must be preheated at 250-350℃.
To improve the performance, the S and P content in the welding materials should be limited, the Si content should be controlled (≤0.30%), and the C content should be reduced. A small amount of Ti, Al, and Ni can be added to refine the grain and reduce hardenability.
Some data shows that adding Nb content (up to about 0.8%) to the welding materials can obtain a single-phase ferrite structure. In CO2 welding wire, Ti and Mn elements should be added to achieve the purpose of deoxidation.
Martensitic stainless steel can also use austenitic stainless steel welding materials. At this time, the influence of base metal dilution on the composition of the weld metal must be considered. By appropriate Cr and Ni content, the formation of martensite structure in the weld metal can be avoided. For example, A312 (E309Mo) welding materials can be used to weld 1Cr13 martensitic steel.
For ferritic stainless steel, it is usually welded with welding materials that are the same as the base material. However, the ferrite structure of the weld is coarse and has poor toughness. The microstructure of the quenched ferrite can be improved by increasing the Nb content in the welding materials.
Meanwhile, heat treatment can be used to improve the toughness of the weld metal. For ferritic stainless steel that cannot be heat treated after welding, pure austenitic welding materials can also be used to obtain welded joints with comprehensive properties.
The welding between low carbon steel and low alloy steel, which both belong to ordinary ferritic steel, as well as the welding between different low-alloy steels, belongs to the welding of same material different steel.
For welding such steels, welding materials are chosen based on the lower grade material, referring to either lower strength level or lower alloy element content, in order to ensure that the weld metallurgical properties can meet the requirements of lower-grade materials.
The selection of lower-grade material also provides better welding performance at a relatively cheaper price, which is beneficial for reducing manufacturing costs.
For instance, when welding same material different steel for 20# steel, SA106 carbon steel, 16Mn, 19Mn6, 15MnMoV, BHW35 and other low alloy steels, the welding materials used are completely identical to those used for welding low carbon steel itself.
The corresponding welding materials for manual arc welding, submerged arc welding, and gas shielding welding are J507, H08MnA+HJ431, and H08Mn2Si, respectively.
Welding of Low Alloy Steel Heat-Resistant Steel and Medium Alloy Steel Heat-Resistant Steel
Due to the discontinuity of the chemical composition of the weld seam in same material different steel, there will be a corresponding discontinuity in performance. If this discontinuity significantly affects usage performance, then welding materials cannot be selected based on low-grade principles.
For example, when welding SA213-T91 and SA213-T22 materials, choosing 2.25Cr-1Mo welding materials for welding according to the usual lower grade principle would result in severe carbon enrichment and decarburization near the T91 base metal of the fusion line on the T91 side.
This is because T91 contains about 9% chromium, while the 2.25Cr-1Mo weld wire contains about 2.25% carbon.
After post-weld annealing treatment, the chromium content in the heat-affected zone on the T91 side is much higher than that on the weld seam side, causing a large amount of carbon to migrate towards the base metal and resulting in carbon enrichment layers, which increase hardness and cause an even harder microstructure.
Conversely, the weld seam side suffers from severe decarburization, with lower hardness and softer microstructure, leading to degradation of the joint’s performance.
If 9Cr-1Mo welding material is chosen, the weld seam on the T22 side will experience carbon enrichment and decarburization of the base material. It should be noted that when components with such chemical composition discontinuities operate at high temperatures, carbon migration continues for a long time, severely deteriorating the joint’s performance and causing operational failures.
Studies have shown that to avoid or reduce the above phenomena, welding materials with intermediate chemical compositions of 5Cr-1Mo can be used for welding, or carbide stabilizing elements such as Nb and V can be added to the welding materials to solidify the carbon element and reduce the occurrence of carbon deviation.
In preliminary experiments conducted by a domestic company, the use of T91 welding materials containing Nb and V, such as CM-9cb, TGS-9cb, and MGS-9cb, for welding the above same material different steel has yielded good results.
When welding dissimilar steel joints of carbon steel, low alloy steel, and austenitic stainless steel, the selection of welding materials should be based on the joint’s working temperature and stress conditions.
For dissimilar steel joints that bear pressure and operate at temperatures below 315°C, welding materials with high Cr and Ni alloy content in austenitic stainless steel can be used. Based on the chemical composition of the carbon steel (alloy steel) and austenitic steel, as well as the size of the fusion ratio, suitable welding materials of austenitic stainless steel with appropriate Cr and Ni contents are selected according to a certain nickel equivalent and chromium equivalent structure diagram to avoid the formation of martensite in large amounts in the weld.
Of course, near the fusion line of the carbon steel or low alloy steel, small martensitic zones may occur. By reducing the carbon content of the welding material, the martensitic structure can become low-carbon martensite with better plasticity, which can ensure good performance of the joint.
For dissimilar steel joints that bear pressure and operate at temperatures above 315°C, nickel-based welding materials should be used. For example, ECrNiFe-2, ERCrNiFe-3, etc. The main reason is that using ordinary austenitic stainless steel welding materials will cause the following problems:
a) Due to the significant difference in the coefficient of thermal expansion between ferrite and austenite, thermal stress and thermal fatigue damage may occur during high-temperature operation.
b) Due to the large difference in alloy element content, severe decarburization and carbon enrichment layers may occur in the welded joint under high temperature operation, leading to deterioration of high-temperature performance.
c) Due to the martensite zone structure near the fusion line, the weld’s local microstructure becomes quenched and hardened.
Using nickel-based welding materials can avoid the above phenomena. This is because:
a) The coefficient of thermal expansion of nickel-based materials is between that of ferrite and austenite.
b) Nickel-based materials will not cause decarburization or carbon enrichment in the welded joint.
c) Nickel-based materials will not produce martensite structure during welding.
This greatly improves the high-temperature performance of the joint.
However, for non-pressure-bearing welded joints operating at high temperatures, although the use of nickel-based electrodes can meet performance requirements, the manufacturing cost is expensive, and there is no need for their use.
Other cheaper welding materials can also achieve the same purpose. Through a large number of experimental studies, foreign countries have found that for non-pressure-bearing fillet welds in boiler manufacturing, when the pipe is made of carbon steel or low alloy steel and the attachment is made of austenitic stainless steel, welding materials should be selected according to lower-grade principles.
For example, when welding SA210C pipes and SA240-304 attachments, AWS E7018-A1 (GB E5018-A1) can be used for manual arc welding, and MGS-M or TGS-M (KOBE welding materials) can be used for gas shielded welding instead of using austenitic stainless steel welding materials.
The main reason is that using austenitic stainless steel welding material will produce martensite zone near the fusion line on the pipe side, and if cracks occur on the pipe side during operation, it will cause pipe leakage. However, using ordinary low-grade welding materials will produce martensite zones near the fusion line on the attachment side. Even if cracks occur, they will not harm the pipe on the attachment side.
Conversely, when the pipe is made of austenitic stainless steel and the attachment is made of low carbon steel or low alloy steel, E309Mo(L) welding material should be used to make the martensite zone occur near the fusion line on the attachment side.
These principles have been applied in the production of 300,000 kW and 600,000 kW heating surface pipes and have been officially applied in the production of 200,000 kW heating surface pipes.